Field trip 2: second day
Deformation and metamorphism of the Tenda Massif and sourrounding Areas
Intinerary: S.Florent, Cava Fontana Morello, F.Poragghia, Fornali, Calaverte, S.Florent
The Tenda Massif
The Tenda Massif forms an elongated culmination of continental-derived metamorphic rocks interposed between the western “authochtonous” and “parauthochtonous” units (plus the Upper nappes of the Balagne) and the eastern “Schistes Lustres” composite nappe system. The Tenda Massif, exposed for nearly 200km2 from S. Florent-Ile Rousse southward to Ponte Leccia (Fig. 2.10) is formed by two major tectonic units: the Cima delle Forchie unit, exposed in the north-west side of the massif, and the Tenda unit (Nardi et al., 1978; Durand Delga 1978; Rossi et al., 2001).
Figure 2.10. Geological map of the Tenda massif
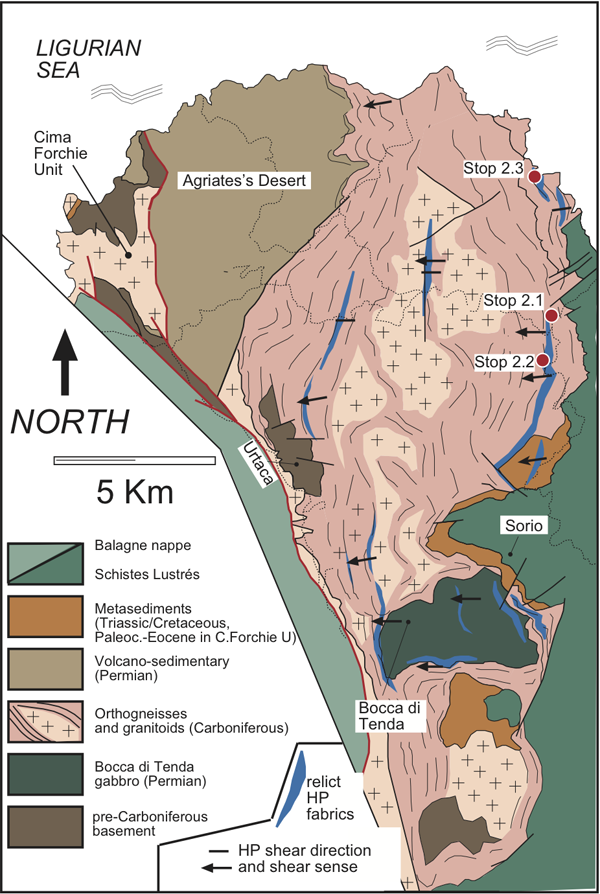
Geological map of the Tenda massif with location of relicts of HO fabrcs. Map modified after Molli and Tribuzio (2004) and based on: Delcey and Meunier (1966); Nardi et al. (1978); Jourdan (1988); Dallan et al. (1983); Rossi et al. (1994); Dallan et al. (1995); Rossi et al. (2001).
The latter is characterized by Palaeozoic granitoids (mainly amphibole/biotite granodiorites and leuco-monzogranites), which are 280-300 My old (Rossi et al., 1994), intruded in the southern part by a gabbroic complex (the Bocca di Tenda gabbro). This gabbroic complex shows well preserved magmatic features and is dated at 274±4 My (Ohnestetter & Rossi 1985). It consists mainly of olivin-gabbronorites, gabbronorites and horneblende-bearing diorites/tonalites (Ohnestetter & Rossi 1985; Rossi et al., 2001; Tribuzio et al. 2001). Granitoids and gabbros are crosscut by doleritic dykes and peralkaline rhyolites both showing chilled margins against the host rocks (Tribuzio et al. 2001).
Remnants of a pre-Carboniferous basement are locally described in the southern and in the northern-western side of the massif (Delcey & Meunier 1966; Nardi et al. 1975; Jourdan 1988; Rossi et al. 1999; Rossi et al., 1994; Rossi et al., 2001). The basement rocks are associated with a Permian volcano-sedimentary cover well exposed in the northern part of the massif (Agriates’s Desert). A reduced Mesozoic metasedimentary cover (Durand Delga 1984; Mattauer et al. 1981; Rossi et al., 2001) crops out in the eastern and southern parts of the massif. These sediments are mainly represented by quarzites and micaceous arkoses (Trias), dolomitic marble and marble (Jurassic) and polygenic metaconglomerates principally formed by basement-derived debris with subordinate carbonatic clasts. These conglomerates are considered as Cretaceous or Cretaceous to Eocene in age (Durand Delga, 1984; Rossi et al., 2001 and references therein).
The Tenda Massif (Fig. 2 and Fig. 2.10) forms a large-scale antiform with an amplitude of ~20km in the north, between S.Florent and Ostriconi, and less than 5km at the southern periclinal termination north of Ponte Leccia. The geometry of this elongated dome is partially related to the activity of a major system of wrench faults (mainly post-Eocene and pre-Burdigalian in age) described at the western boundary of the Tenda Massif (Central Corsica Fault zone of Maluski et al. 1973; Waters, 1990; Rossi et al., 2001; Molli, 2008) and to a late phase of antiformal stacking occurring during the Late Eocene.
Structures related to a polyphase deformation history can be observed in cover rocks of the Tenda unit, where at least two generations of isoclinal folds are overprinted by a late phase of open to kink folds. On the contrary, basement rocks commonly exhibit a simpler structural pattern. More specifically, a regional-scale main foliation wrapping around undeformed domains (meter to kilometer in scale e.g. Casta granodiorite of Rossi et al., 1994), can be recognized in granitoids. The main foliation is generally characterized by greenschist facies assemblages, although relict domains preserving pre-greenschist structures can be found (see below). On the basis of shear directions, overprinting relationships and petrologic data, the structures formed in greenschist facies metamorphic conditions have been subdivided into two groups, interpreted as developed at different structural levels (Molli and Tribuzio, 2004; Molli et al., 2006).
The younger structures (D3) are characterised by localized zones of deformation from centimeter to meter in size, forming shear band systems associated with northeast/southwest trending stretching and mineral lineations (Fig. 2.11) and top-to-northeast shear sense indicators. These structures can be easily recognized toward the eastern border of the Tenda Massif, where they were firstly described by Waters (1990), Jolivet et al. (1990) and later on by Daniel et al. (1996), Egger and Pinaud, (1998) and Guyedan et al. (2003). D3 structures are also present inside the massif, where conjugate shear zones were described by Molli and Tribuzio (2004) and interpreted as related to partitioned coaxial strain and vertical shortening far from the eastern border of the Tenda Massif.
Figure 2.11. Equal area projection plots
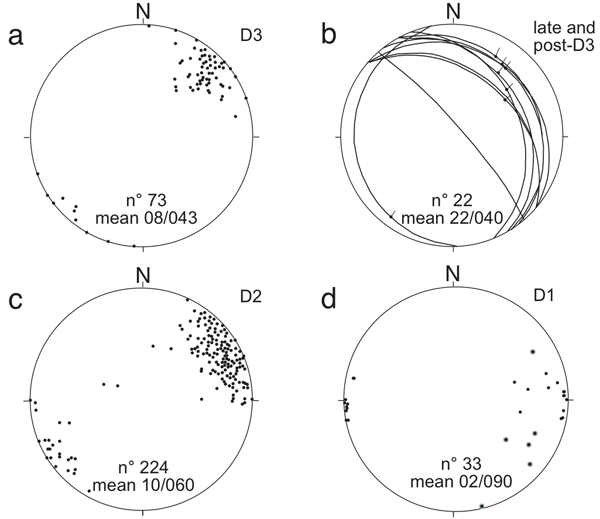
Equal area projection plots (lower hemisphere) of: (a) stretching/mineral lineation of D3; (b) late- and post-D3, great circles for shear bands and faults with sense of movement and slicklines; (c) D2 and (d) D1 stretching/mineral lineations. In (d) black dots and mean trend refer to lineation in D1domains unaffected by D2/D3 overprint, whereas black dots with grey rims refer to D1 lineation in relicts domains within D2 or D3 dominant fabrics. After Molli et al. (2006).
D3 fabrics in granitoids are associated with celadonite-poor phengite (Si = 3.2-3.3 apfu, Fig. 2.12) + epidote + albite + quartz (± calcite ± chlorite) assemblages. The dolerite dykes developed chlorite + albite + epidote (± quartz ± calcite ± phengite). Metabasites are thus characterized by the absence of amphibole and can be related to the “lowermost greenschist facies” (Spear, 1993). These mineral assemblages indicate that the D3 deformation occurred at temperatures of 300-400°C and pressure conditions lower than 0.5 GPa.
Figure 2.12. Phengite compositions
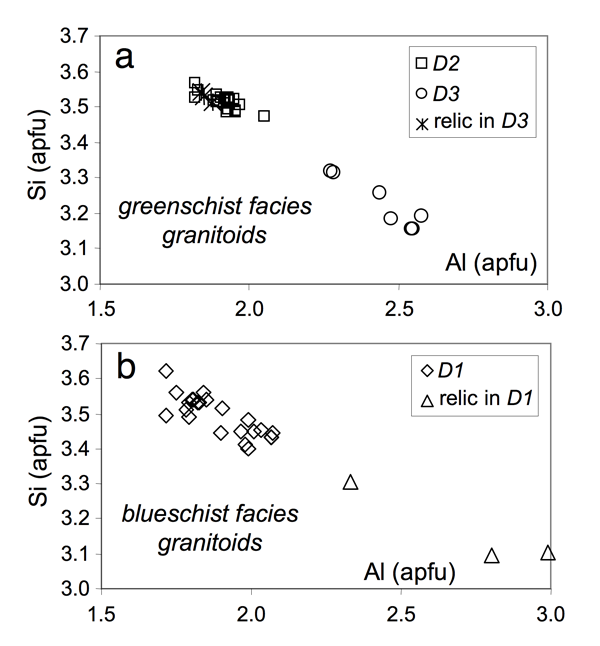
Phengite compositions from metagranitoids deformed under greenschist (a); and blueschist (b) facies conditions; Si vs. Al tot (atoms per formula unit, apfu). D1, D2 and D3 = phengites related to D1, D2 and D3 fabrics, respectively.
Meter- to pluridecameter D3 open to close folds associated with sub-horizontal to moderately dipping crenulation cleavage can be locally observed. They commonly show fold axis sub-parallel to the stretching lineation of the surrounding D3 shear zones. Semi-brittle shear bands and cataclasite-bearing fault zones (late- and post-D3 structures) geometrically and kinematically coherent with D3 structures are locally present (Fig. 2.11). They are interpreted as related to a progressive deformation at lower temperature conditions in the uppermost crust. D3 structures therefore evolved toward shallower structural levels with late- and post-D3 structures.
The main fabric along the eastern border of the Tenda Massif (as well as inside of it) can be referred to the D2 stage of greenschist facies deformation. This fabric shows stretching and mineral lineations (Fig. 2.11) oriented east-northeast/west-southwest (mean attitude toward 60°). Shear sense indicators such as asymmetric porphyroclasts and shear band systems of C- and C’-types show both top-to-west (southwest) and predominant top-to-east (northeast) kinematics (Fig. 2.11). D2 structures in granitoids are associated with celadonite-rich phengite (Si ~3.5 apfu, Fig. 2.12) + epidote + albite + quartz (± calcite ± chlorite ± actinolite) assemblages.
The heterogeneous deformation pattern that characterizes the Tenda unit allowed us to recognize and analyse domains of mylonitic orthogneisses and mylonitized doleritic and peralkaline rhyolitic dykes with a well preserved HP/LT fabric (D1 structures, Fig. 2.11) unaffected by greenschist facies static and/or dynamic retrogression.
The occurrence in the Tenda unit of lithotypes showing large compositional variations, allowed to estimate the peak metamorphic conditions (Fig. 2.13; 2.14, more details in Tribuzio and Giacomini 2002 see also Maggi et al., 2011 and stop 2.1). Evolved rocks of the gabbroic sequence (quartz-diorite/tonalites), basalt doleritic dykes and granitoids are characterized by epidote-blueschist assemblages, as they show the coexistence of riebeckite/ferroglaucophane, epidote, celadonite-rich phengite (Si= 3.5-3.6 apfu) and albite. The peralkaline rhyolites develop a metamorphic paragenesis, defined by jadeite-bearing (up to 46 mol%) aegirine, riebeckite, celadonite-rich phegites (Si= 3.5-3.6 apfu), quartz, albite and K-feldspar. The Mg-rich lithotypes (olivine gabbronorites to gabbronorites) are characterized by the absence of blue amphibole. In particular, we have found that the gabbronorites develop a mineral association that can be related to the epidote-amphibolite facies, as they display the coexistence of Al-poor horneblende (Al2O3 < 6.6 wt%), albite, epidote and phengite. These multisystem assemblages allow the peak P-T metamorphic conditions to be constrained at 1.0 ± 0.1 GPa and 450 ± 50°C (Tribuzio & Giacomini, 2002; Molli & Tribuzio, 2004). These values attest to a geothermal gradient (dT/dP) of 10/13°C km-1, thus suggesting a subduction-related tectonic setting (Tribuzio & Giacomini 2002 Molli & Tribuzio, 2004; Molli et al., 2006).
Figure 2.13. HP/LT mineral assemblages
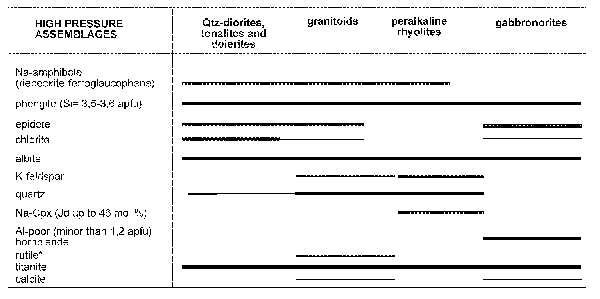
Significant HP/LT mineral assemblages in different rock types in the Tenda unit (thin lines represent mineral that are only locally present) (modified after Molli and Tribuzio, 2004).
Moreover, the D1 relict structural domains are characterized by E-W oriented stretching and/or mineral lineations defined by Na-amphibole and are associated with a top-to-the-west shear sense. These kinematic indicators, observed at the kilometric distance, for their constant attitude at regionale scale were considered relevant for the deep deformation and history of the Tenda unit, which has to be related with a east-dipping subduction (e.g. Mattauer et al., 1981; Cohen et al., 1981; Gibbson & Horack, 1984; Warburtoun, 1986; Waters, 1990; Malavieille et al., 1998; Marroni & Pandolfi, 2003; Molli & Malavieille, 2010 and reference therein).
At its eastern border the Tenda unit is in contact with a typical ophiolitic unit, consisting of serpentinites, metabasites and a metasedimentary cover. The latter shows significant variations from the north, where the metabasites are stratigraphically followed by quartzitic-micaschists and calcschists, to the south, near S. Pietro di Tenda, at the south-eastern edge of the Tenda massif where pure quarzites, marbles and calcschists crop out.
The peak metamorphic assemblages in the metabasites derived from lava flows are characterized by Na-amphibole + albite + chlorite + epidote + phengite (± calcite). Therefore, the metabasalts display typical epidote-blueschist facies (Evans, 1990) assemblages, similar to those observed in the metadolerites within the Tenda orthogneisses and in the metadiorites from the Bocca di Tenda gabbroic sequence (Tribuzio and Giacomini, 2002). In the ophiolite unit, blueschist facies metabasites derived from Fe-Ti-rich gabbroic protoliths have also been found, for instance at Monte S. Angelo (kilometric coordinate 566.9/249). These rocks commonly preserve mineral relicts of the igneous paragenesis (i.e. ilmenite and Ca-clinopyroxene), (Fig. 2.14) and display a peak metamorphic assemblage with Na-amphibole + aegirine + albite + chlorite. Ophiolitic Mg-Al-rich gabbros are also found and are characterised by the absence of Na-amphibole, similar to the gabbronorites from the Bocca di Tenda gabbroic sequence (Tribuzio and Giacomini, 2002; Molli and Tribuzio, 2004). Remarkably, the chemical composition of Na-amphiboles from blueschist facies metabasites of the Tenda Massif and from the ophiolitic unit are similar (Fig. 2.14), suggesting that both unitsunderwent similar peak metamorphic conditions. This conclusion has been confirmed recently with Raman Spectroscopy on Carbonaceous Material (Vitale Brovarone et al., submitted).
Figure 2.14. Amphibole compositions from Tenda microgabbro
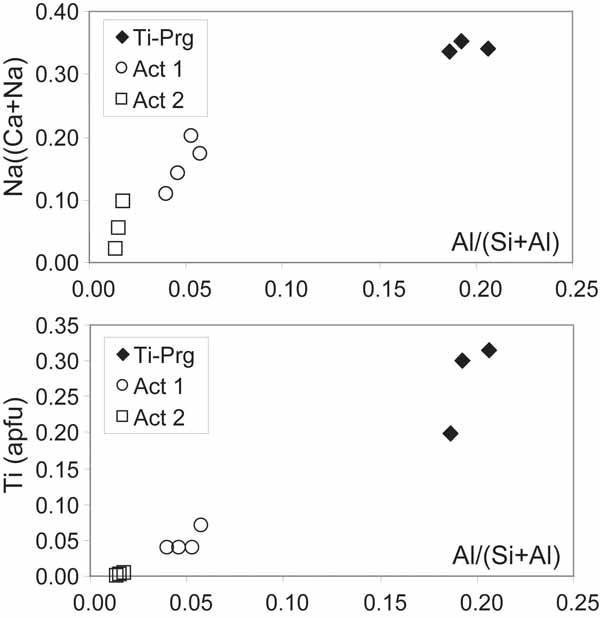
Amphibole compositions from Tenda microgabbro with greenschist facies metamorphism, involved in the D2 deformation event. Na/(Ca + Na) vs. Al/(Si + Al) and Ti vs. Al/(Si + Al) in apfu. Ti-Prg = Ti-pargasite of igneous origin; Act 1 = actinolite in coronas around Ti-Prg; Act 2 = actinolite in top-to-west shear band systems (D2 event).
Blueschist facies assemblages in metabasites are commonly partially overprinted by greenschist facies parageneses, with development of chlorite + albite + epidote (± phengite ± calcite ± actinolite). A widespread re-equilibration under greenschist facies conditions is common in the ophiolitic metasediments. The ophiolitic unit is characterised by distributed deformation associated with pervasive folding from map to thin section scale. Two generations of isoclinal folds (D1 and D2) are associated with the two main stages of metamorphism (under blueschist and greenschist facies conditions, respectively), whereas later stages of folding are characterised by sub-horizontal to sub-vertical axial-planar disjunctive crenulation cleavage and kink bands (D3 and late-D3).
Figure 2.15 reports the P-T-t-d evolution of the units in contact along the eastern border of the Tenda Massif. Both oceanic and continental (Tenda) units are characterised by peak metamorphic conditions of ~450°C and 1.0 GPa, which were attained during D1 deformation. D2 deformation and structures can be related to the exhumation history, which started at HP/LT metamorphic conditions and evolved towards lower pressure, when the early greenschist facies assemblages formed. The following D3 structures formed at T = 300-400°C and P<0.5 GPa and were overprinted at shallow structural levels by the late- and post-D3 semibrittle and brittle structures. D3 and late- to post-D3 structures are localized and individual structures accommodated little displacement (from millimeters to meters). In addition, their impact on the overall crustal geometry is negligible, as suggested by the undisturbed D2 large scale folds at the contact between oceanic and continental units. Recently acquired structural and petrologic data (Maggi et al., 2011 and Maggi, 2011) largely confirmed the structural history described above. Furthermore, a U/Pb TIMS study on syn-kinematic rutile in D1 phyllonites yelded an age of 48 ± 18 Ma (MSWD 7.3), while an intercept at 54 ± 8 Ma (MSWD = 48) was obtained from coexisting acmite-phengite and ox/sulph coatings.
Figure 2.15. Pressure-Temperature-deformation
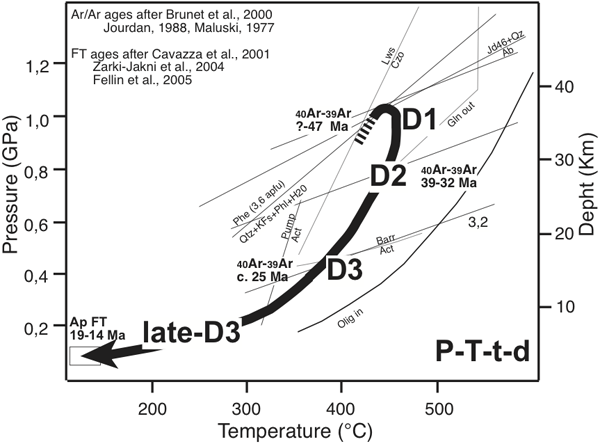
Pressure-Temperature-deformation path of continental Tenda and ophiolitic units justapoxed across the eastern border of Tenda Massif after Molli et al. (2006). Gln out taken from Maresch (1977), the reaction pumpellyite + chlorite = actinolite + epidote after Liou et al. (1983), lawsonite-clinozoisite transition after Barnicoat and Fry (1986), the lower stability limit of barroisite from Ernst (1979), oligoclase-in reaction from Maruyama et al. (1983), the reaction curve for Na-clinopyroxene (Jd46) + quartz = albite was calculated with the 3.1 version of THERMOCALC program (Holland and Powell, 1998, and references therein). The reaction curve for Mg-phengite (Si = 3.6 apfu) = quartz + K-feldspar+ phlogopite + H2O) is after Massonne and Szpurska (1997). Ar/Ar data after Brunet et al. (2000), Jourdan (1988) and Maluski (1977). Fission track apatite ages (Ap FT) after Cavazza et al., 2001; Zarki- Janki et al. (2004) and Fellin et al. (2005). Note that new data of Maggi et al. 2011 suggest for the HP/LT event an age between 55 and 48 Ma.
Day Two Itinerary
Stop 2.1:
Locality: Fontana Morello Quarry (UTM 32T 522329 E 4722712)
Themes: Heterogeneous strain in the ETSZ, gneissic fabrics and the Acmite-Rutile-bearing phyllonites
A panoramic view of the eastern margin of Tenda Massif will be shown to illustrate the heterogeneous strain in this areaThe analysis of the Fontana Morello Quarry will allow to describe the range of fabrics and mineral assemblages of the ETSZ More specifically, this stop aims at illustrating (i) the heterogeneous distribution of shear strain within the ETSZ, with zones of localized ductile-to-brittle shearing (SZ) that wrap around lens-shaped massive bodies with prominent gneissic fabric (ML); (ii) the effects of the intense fluid-rock interaction along the ETSZ with associated changes in textures and mineralogy; and (iii) the high-pressure (Na-amphibole/acmite-rutile bearing) phyllonitic shear zones.
The NW-SE striking main foliation in the ML, which generally dips to the NE at a shallow angle, is concordant with that in the overlying Schistes Lustrés. The ML mineralogy consists of a high-variance assemblage made of quartz + phengitic muscovite + epidote and (relict) feldspar (Fe-oxides, zircon and allanite as accessory phases). The SZ mineralogy is invariably dominated by Si-rich (Si4+ = 3.5-3.7 apfu) phengite (40-60 vol%), modally abundant quartz (30-50 vol%), albite (10-20 vol%) epidote (5-10 vol%) and microcline (5-10 vol%). Locally, Na-amphibole (10-20 vol%) also occurs in the SZ assemblage to form thin (up to 1 meter thick) dark mylonitic levels. Collectively, due to the predominance of phengite in the shear zone assemblages (locally > 60%), the SZ consists of phyllonites. with stretching lineations striking WSW-ENE to E-W. quartz-phengite-albite characterize ML and whereas Na-amphibole-quartz-albite-phengite may be found in SZ. Kinematic indicators are abundant within the two main rock types and invariably point to top-to-the-W/NW sense of shear.
At about 100 meters below the main contact, a 10 cm thick phyllonitic layer (Fig. 2.16b) contains the high-pressure association rutile-acmite-phengite-albite-epidote-Na-amphibole. Transition from the gneissic host rocks to the shear zone is marked by a green alteration halo made of phengite aggregates (Fig. 2.16b). Kinematic indicators both at the meso- and micro-scale, systematically indicate top-to-the-west ductile shear sense (Figs. 2.16c-d). Acmite and rutile form porphyroblasts along the main foliation; acmite grains host inclusions of Na-amphibole and are rimmed by retrograde biotite (Figs. 2.16e-f). The modal composition consists of phengite (>60% vol.), acmite (25% vol.), rutile (5% vol.), quartz plus accessory phases (albite + microcline + epidote + relict zircon; 10% vol.). Microprobe data and BSE images indicate a rather homogeneous composition both for phengite (3.6-3.7 Si4+ apfu) and acmite (average composition Jd17Di9Hed10Px0.05) grains. On the other hand, phengite shows a strong core-to-rim chemical zoning (Si4+ content ranging from 3.2 to 3.6 apfu, respectively) (Fig. 2.16g).
Figure 2.16. Stop 2.1
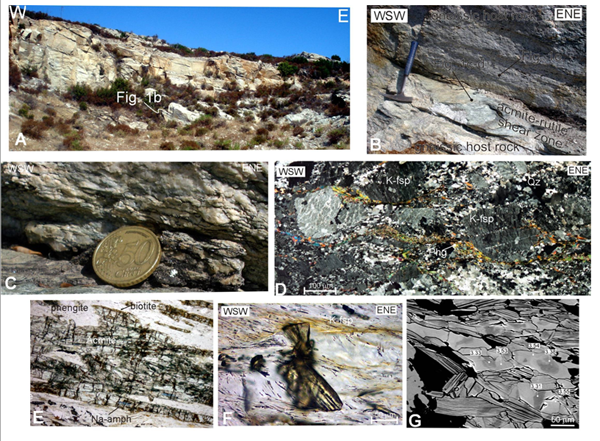
(a) Panoramic view of the Stop 2.1, with indicated the acmite-rutile phyllonites. The dominant lithological type consists of massive gneisses with main foliation dipping shallowly towards the E; (b) The acmite-rutile phyllonite in the field; (c) Kinematic indicators in the host rocks (exposure normal to foliation and parallel to the stretching direction). Shear sense id dominantly provide by S-C fabrics and s-type porphyroclasts made of relict K-feldspar; (d ) Kinematic indicators at the thin section scale (section cut normal to foliation and parallel to the stretching direction); (e) Porphyroblastic acmite crystals, hosting Na-amphibole as inclusion and rimmed by biotite; (f) Porphyroblastic rutile in the phyllonite matrix; (g) - BSE images showing the phengite chemical zoning (numbers refer to the measured Si4+ a.p.f.u).
Stop 2.2
Locality: Fontana Poragghia (UTM 32T 521706E 4720892)
Themes: blueschist orthogneiss and overprinting fabrics.
Widespread relicts of pre-greenschist facies assemblages and structures (D1) can be observed all along the eastern border of the Tenda Massif as shown in Fig. 2.5. An easily accessible exposure will be visited along the D62 (kilometric coordinate 567.5/262.07), near the Fontana Porraghia site (Fig. 2.17). Here, orthogneiss and metadolerite dykes hosting HP/LT fabrics will be observed and the styles of GS structural overprinting analysed.
Figure 2.17. Fontana di Porragghia
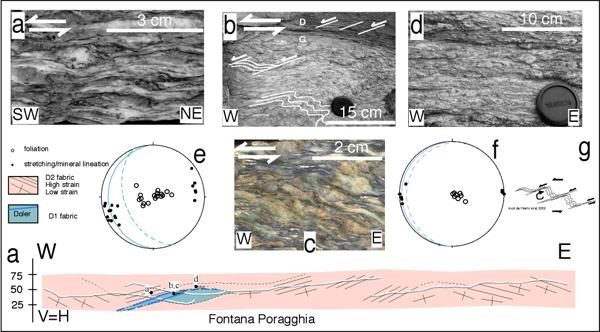
(a) Cross-section of Fontana di Porragghia and surroundings showing D1 shear zone relicts and D2 foliation with gradient of D2 strain; equal area projection plots (lower hemisphere) of pole of foliations, great circles of shear bands and stretching lineations D2 (e) and for D1 (f) deformations; (a) D2 fabric with well developed top-to-southwest shear bands; (b) contact between mylonitized dolerites (D) with top-to-west shear bands and granitic mylonites (G) with top-to-west shear bands and centimeter-scale backfolds; (e) D2 crenulation with greenschist retrogression of former D1 fabric in the microlithons; (g) schematic diagram showing development of east-vergent folds between shear band systems associated with top-to-west shearing, on the basis of Harris et al. (2002).
In Fontana di Poragghia, the orthogneisses locally show a main foliation defined by Na-amphibole+ celadonite-rich phengite (Si ~3.5 apfu, Fig. 2.12) + epidote + albite + quartz (± chlorite ± K-feldspar). White mica cores locally preserve high-celadonite (Si = 3.1-3.3 apfu) and Ti-poor compositions, which can be attributed to a low-pressure stage of the prograde path.
The blueschist-facies fabric is characterized by mineral (Na-amphibole) and stretching (quartz aggregates) lineations striking east/west (mean toward 268°), with top-to-west kinematics, defined by σ-type asymmetric porphyroclast and widespread shear bands in metadolerites (Fig. 2.17). Centimeter to decimeter-scale E-vergent folds deforming the blueschist foliation can be observed in orthogneisses located inbetween mylonitic metadolerites. The folded Na-amphibole fabric is not retrogressed to greenschist-facies assemblages, thus allowing us to interpret the folds as related to the back-rotation of the blueschist foliation in a progressive history of top-west shearing. This event possibly occurred during the development of shear bands in metadolerites, in the latest stages of blueschist shearing. Fig. 2.17g illustrates fold/shear zone relationships as observed from decimeter to microscale and their interpretation according to the model described by Harris et al. (2002).
The blueschist foliation grades to orthogneisses characterised by greenschist facies assemblages, and the transition shows a spatial variability normal to the strike. Toward the west, the blueschist facies foliation coplanarly grades to a greenschist foliation characterised by a well-developed lineation of stretching and mineral type oriented toward 248° southwest (Fig. 2.17). In this domain, asymmetric porphyroclast systems and well-developed shear bands indicate top-to-the-southwest kinematics (Fig. 2.17). East of Fontana Porraghia, the transition to the greenschist foliation occurs through a decameter thick domain of strongly crenulated orthogneisses. This domain shows the partial to total breakdown of blueschist facies Na-amphibole (Fig. 2.17).
Stop 2.3:
Locality: Walking along the coast from Fornali to Calaverte (UTM 32T 521788 E 4727588)
Themes: The ETSZ and the geometries of the contact between the Tenda and the ophiolitic unit.
This stop will involve a walk towards the north, along the coast from Fornali lighthouse to Calaverte bay. During this walk, it will be possible to observe structural geometries within the ETSZ and the relationship between the Tenda crystalline massif and the ophiolitic unit.
As shown in Fig. 2.18, between St.Florent and the Fornali lighthouse, the ophiolitic unit, consisting of metabasites and calcschists, are overlain by orthogneisses and a mylonitised volcano-sedimentary cover, both Tenda-derived. According to the interpretations of Waters (1990) and Dallan and Puccinelli (1995), the orthogneisses are not Tenda-related and form tectonically interleaved continental–derived slices similar to those found east of St.Florent (Unità degli ortogniess superiori di Dallan & Puccinelli, 1995; Fornali nappe of Waters, 1990). Rossi et al., (1994) and (2001) proposed a similar interprerpretation, although the tectonic slices were condidered as Tenda-derived.
Figure 2.18. Geologic sketch map of the area west of S. Florent
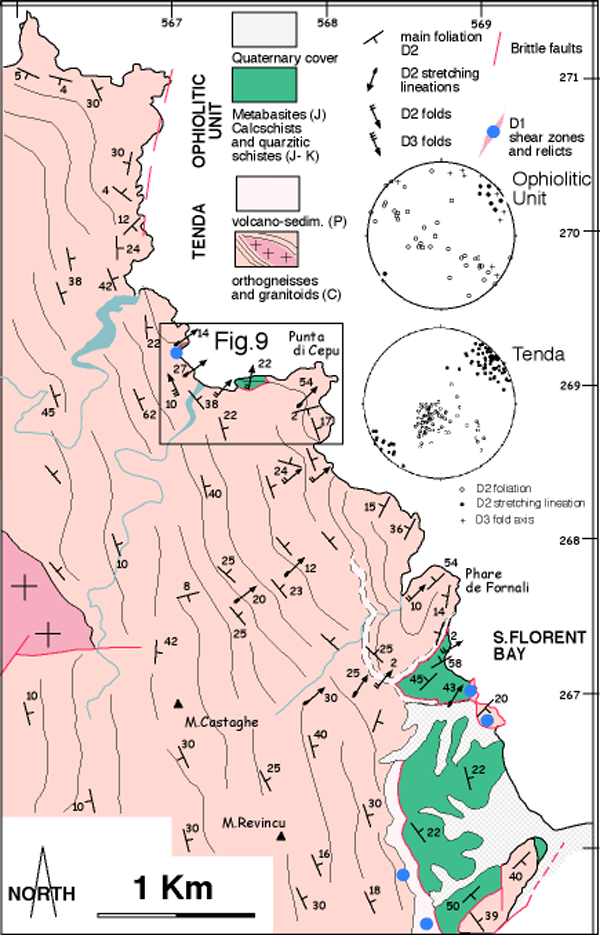
Geologic sketch map of the area west of S. Florent, with inset of equal area projection plots (lower hemisphere) of pole of foliation, stretching/mineral lineation, and fold axes of D2 structures in the Tenda and ophiolitic units. Rectangle indicates the location of Fig. 2.19.
Figure 2.19. Geological map of the Calaverte bay
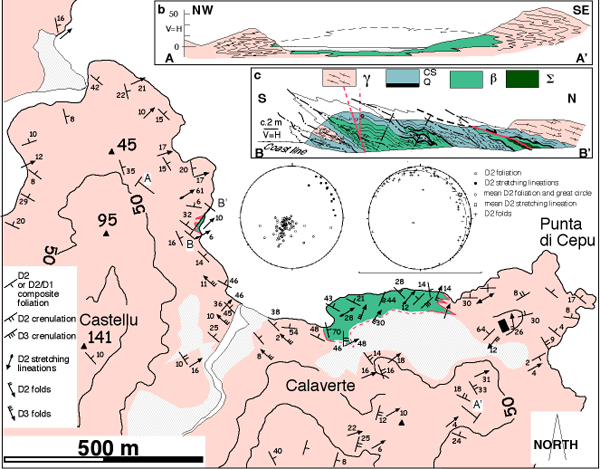
Geological map of the Calaverte bay and surroundings, with inset of equal area projection plots (lower hemisphere) of pole of foliation, stretching/mineral lineation, and fold axes of D2 structures; (b) geological cross section of the Calaverte area, traced normal to the trend of D2 stretching lineation, with the interpretation of the ophiolite unit exposure within the Tenda orthogneiss as lateral termination of D2 sheath-fold; (c) schematic cross-section of north-west exposure of ophiolitic unit along the coast with observable structures. γ are orthogneiss; CS and Q are calcschists and quarztites; β, metabasic rocks and Σ actinolite-tremolite schists.
The geological maps of Fig. 2.18, 2.19 describe in some details the geometry of the contact between the Tenda and the ophiolite unit west of S.Florent. Key exposures are located to the south of the Fornali lighthouse, between Fornali and Punta Cepu, where the ortogneiss and the mylonitized Tenda-derived cover are affected by pervasive isoclinal folds at different scales, withstrongly curved intersection lineations on foliation planes. The axial planar foliation of the isoclinal folds shows greenschist facies assemblages with phengite composition (Si = 3.4-3.5 apfu) similar to D2 structures in other parts of the Tenda.
Further north in the Calaverte bay (Fig. 2.19), the ophiolitic unit reappears with a complete litostratigraphic sequence, with actinolite-tremolite schists, metabasites, quartzitic-micaschists and calcschists forming tight folds within the orthogneiss (Fig. 2.19). Meso- and microstructural features show that the axial planar foliation of the folds is associated with greenschist assemblages deforming a previous blueschist fabric. These two foliations (and the associated isoclinal folds D1 and D2) are both deformed by a west-dipping disjunctive crenulation cleavage that is axial planar to metric scale east-vergent open to close folds (D3). Our interpretation for the exposure of the Calaverte Bay is that of the lateral terminations of a strongly non-cylindric recumbent fold (Fig. 2.19). The ophiolitic unit (calcschists and metabasites) is pinched within the orthogneisses, which therefore were more competent during deformation. These structures are considered as being due to the buckling of the original thrust contact (with the ophiolitic unit arranged in an inverted limb of kilometer-scale west vergent isoclinal D1 fold above the Tenda orthogneiss), followed by rotation and stretching of the earlier fold during southwest/northeast directed shearing.
Similar geometries of deformation can be also observed south of S.Pietro di Tenda, around the M.Buggentone area and further south, in the Ponte Leccia surroundings. Therefore, at the scale of the whole eastern border of the Tenda, the contact between the continental Tenda and the ophiolitic units appears tightly folded in large scale recumbent isoclinal non-cylindric D2 folds. This has important implication for the interpretation of the ETSZ which can hardly be interpreted as a core complex of Cordilleran type (Jolivet et al., 1990; Daniel et al., 1996; Gueydan et al., 2003). On the contrary, the Eastern Tenda Fault Zone shows the main tectonic grain related with the deep seated tectonic processes associated with subduction and syn-collision intrawedge-deformation possibly developed during the underplating of more external units. Thus, although extension is commonly invoked to explain exhumation of metamorphic rocks and synchronous enigmatic zones of normal shearing observed in most mountain belts, in many cases, this cannot be the dominant mechanism. Since many years, uplift induced normal sense shear zones and concommitant brittle normal faults (developed at lower depth) are described in both ancient (e.g. the Variscan belt, Pérez-Estaùn et al., 1991) or active mountain belts (e.g. the Taiwan belt, Crespi et al., 1996). Interpretation of such deformation features in the frame of mountain building is still controversial today and proposed models range between end-members involving compressional (convergent) or extensional (divergent) settings. Analog experiments with décollements and erosion (e.g. Konstantinovskaia & Malavieille 2005; Malavieille, 2010) suggest an alternative way to develop crustal scale normal sense shear deformation during continental subduction. Such structures can be the result of the vertical shear induced by strain partitioning within the orogenic wedge (Fig. 2.20). Continuous uplift of underplated crustal units relative to comparatively stable surrounding rocks favors vertical shear and as a consequence a strong stretching and thinning of the formerly stacked tectonic units. At depth these domains are characterized by the development of foliation zones of combined pure and simple shear deformation with a normal sense shearing component. They can evolve to brittle normal faults superimposed on former ductile foliation when reaching upper-crustal domains during synconvergence erosion assisted uplift.
Figure 2.20. Cartoon after Malavieille (2010)
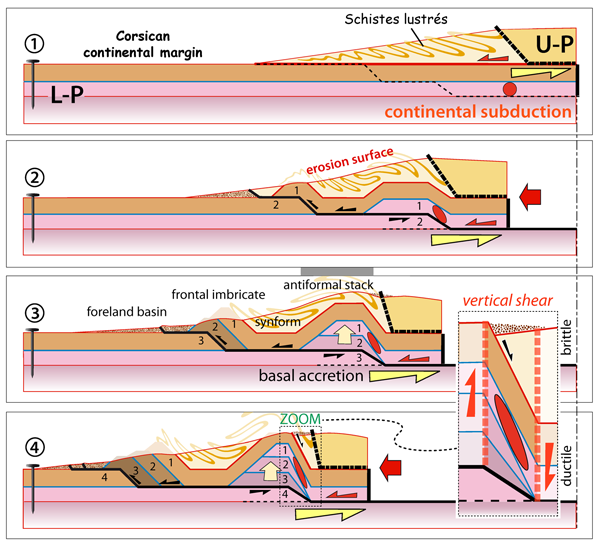
Cartoon after Malavieille (2010) showing deformation partitioning and kinematics of units in a décollement type wedge. Notice the deformation of upper plate (orogenic lid) resting on top of the former refolded décollement. Early folds are suggested to show evolution of U-P geometry. A possible deformation mechanism responsible for vertical shear inducing stretching and thinning of the underplated units is schematized. Red ellipsoids show resulting strain. U-P = pre-structured upper-plate, L-P = basement lower-plate.
Remarkably, across the ETSZ, there is no significant difference in geochronological ages across the eastern border of the Tenda Massif, between footwall and hanging wall of the supposed detachment fault, for both high (40Ar/39Ar on white micas) and low (apatite fission track) temperature systems (Cavazza et al., 2001; Zarki-Janki et al., 2004; Fellin et al., 2005). This agrees with the structural data observable, which indicate a weak (low displacement) extensional-related reactivation of previous oldest and deepest fabric. In addition, the presence of clasts of Tenda-derived granitic mylonites within the lowermost part of the S.Florent sequence (see also Dallan and Puccinelli, 1995; Ferrandini et al., 1998) argues against the interpretation of the Miocene of S.Florent as a supra-detachment extensional basin related to the activity of the East Tenda shear zone at depth, as proposed by Jolivet et al. (1990).