Discussion
The Central Andean terranes and evolution of the Rocas Verdes Basin
A major constraint in the reconstruction of the Southern Andes regards the original extent of the Rocas Verdes Basin. Mafic volcanics, attributed to the Rocas Verdes Basin, are found from the southern tip of Patagonia through to the Sarmiento Complex (Dalziel et al. 1974). These volcanics suggest the Basin did not extend north of approximately 50°N (Fosdick et al. 2011). This observation was incorporated into the Southern Andean tectonic models of Cunningham (1993), Cunningham (1995), Diraison et al. (2000), (Kraemer, 2003), and this paper. In the tectonic models of such studies the Rocas Verdes Basin is a small marginal basin that opens behind an active andesitic island arc during the Cretaceous.
However, a recent study by Vérard et al. (2012) presents a different model, whereby the Rocas Verdes Basin is up to 3000 km wide, and extends from Patagonia to the Central Andes. Vérard et al. (2012) suggest the width of the basin is constrained by the Early Cretaceous collision of cross-Pacific GDUs (a term given to the various geological units that originated in the ‘Pacific magnetic triangular zone’ of Müller et al. (2008)), and terranes rifting from South America during the formation of the Rocas Verdes Basin. However, the data for this collisional event (Thomson and Herve, 2002; Herve and Fanning, 2003; Willner et al. 2004) is derived from work undertaken in the Southern Andes only. However Vérard et al. (2012) have included the rifting of Central Andean terranes (such as Arequipa) off the Andean margin as part of the Cretaceous opening of the Rocas Verdes Basin. We have not applied the same reasoning, and have taken the approach that the Central Andes followed a different evolution to that of the Southern Andes – one that does not involve the Rocas Verdes Basin.
Palaeozoic samples, from the Sierra de Almeida of northern Chile, show discordance from the Gondwanan palaeomagnetic path only for the latest Cambrian-earliest Ordovician (Forsythe et al. 1993). Forsythe et al. (1993) explained this discordance whereby the Arequipa block rotated about a nearby pole but was resutured to Gondawana during the Silurian. Prior to this event Arequipa was rifted during the breakup of Rodinia in the Neoproterozoic (Sempere, 1995), however has remained in situ since this Silurian reaccretion event.
While there is conjecture as to whether the Arequipa and Antofalla terranes are part of the same block (Ramos 2009), there are numerous data advocating that the Antofalla terrane reaccreted to the continental margin by the Late Ordovician (Ramos, 2008 and references therein). The Famatina and Cuyania terranes accreted during the Middle Ordovician, as evidenced by significant deformation during the Famatinian orogeny (Astini et al. 1995; Pankhurst et al. 1998; Quenardelle and Ramos, 1999). Various geochronological and geological data suggest the Chilenia terrane is likely to have accreted during the Late Devonian (Ramos, 2010). These data suggest the Central Andean terranes have remained as a part of South America since their Palaeozoic accretion and/or reaccretion, and thus were not involved in any Mesozoic rifting related to formation of the Rocas Verdes Basin. Subsequently our model follows that of Cunningham (1993), Cunningham (1995), Diraison et al. (2000), and (Kraemer, 2003), whereby the Rocas Verdes Basin is a small marginal basin that opens behind an active island arc.
Crustal deformation in the Central Andes
The model we have developed uses data suggesting that during the Mid-Eocene to Mid-Miocene shortening was focused in the region of the central Bolivian Andes (16°–24°S), with a total shortening ~400 km as presented by Arriagada et al. (2008). This amount of shortening, and the inherent bending of the Bolivian orocline during the Mid-Eocene to Pliocene, may not fully account for the observed total palaeomagnetic rotations of >25° north and south of the Arica Bend for this time period (Arriagada et al. 2008). That said, it should be emphasized that this difference between the observed and predicted palaeomagnetic data is not conclusive, and may simply be a consequence of data resolution. However if one were to examine the implications that the palaeomagnetic data suggest, either the orogen was actually straighter prior bending, or that in situ block rotations have contributed (Figure 4). Beck (1998) proposed that sub-crustal ductile flow is likely to have caused significant local block rotations, and thus would be a plausible mechanism to explain the additional rotations observed within the palaeomagnetic data.
Figure 4. Regional-scale structural kinematics of the Bolivian Orocline, after Randall et al. (1996) and Beck (1998).
![]() |
The strike-slip domino model (starting configuration a., final configuration b.), that occurs as a consequence of oblique convergence, and results in the formation of left-lateral antithetic faulting between the dominant – in this case right-lateral – strike-slip systems. This faulting contributes to oroclinal bending, and additional rotation of fault-bounded blocks as observed by several palaeomagnetic studies. Note that in order for crustal volume to be conserved the deformation zone must lengthen and/or crust thicken.
Dynamics of the Andean subduction zone
The Cretaceous to present Andean subduction zone records a dynamic history with respect to cratonic South America (Figure 5). The Northern Andean subduction system is relatively stable, with the only movement being westward as a result of the addition of crust through accreted terranes. The Central Andean region experiences a large Eocene/Miocene shift subduction zone location as a result of orogenic shortening and orocline formation. Oroclinal formation also has a large influence on subduction in the Southern Andes, with the southern extremity of the system potentially experiencing migration upwards of 600 km relative to South America. This illustrates the potential forces subjected to lithosphere subducting beneath South America. Such forces are likely to be amplified when absolute motion of the overriding plate is considered. Studies analysing tectonic processes occurring along the Andean margin, and its subduction system, must consider the lateral movements of the subduction system through time. Subduction dynamics are likely to have had a large influence on magmatism, metamorphism, mineralisation, and the morphologic evolution of subducting lithosphere. However, it is important to note we have not accounted for subduction erosion of the forearc, a process that is likely to have affected the lateral positioning of the palaeo-subduction zone, and thus subsequent conclusions.
Figure 5. Trench dynamics of the Andean subduction system from 145 Ma to present, relative to stable South America.
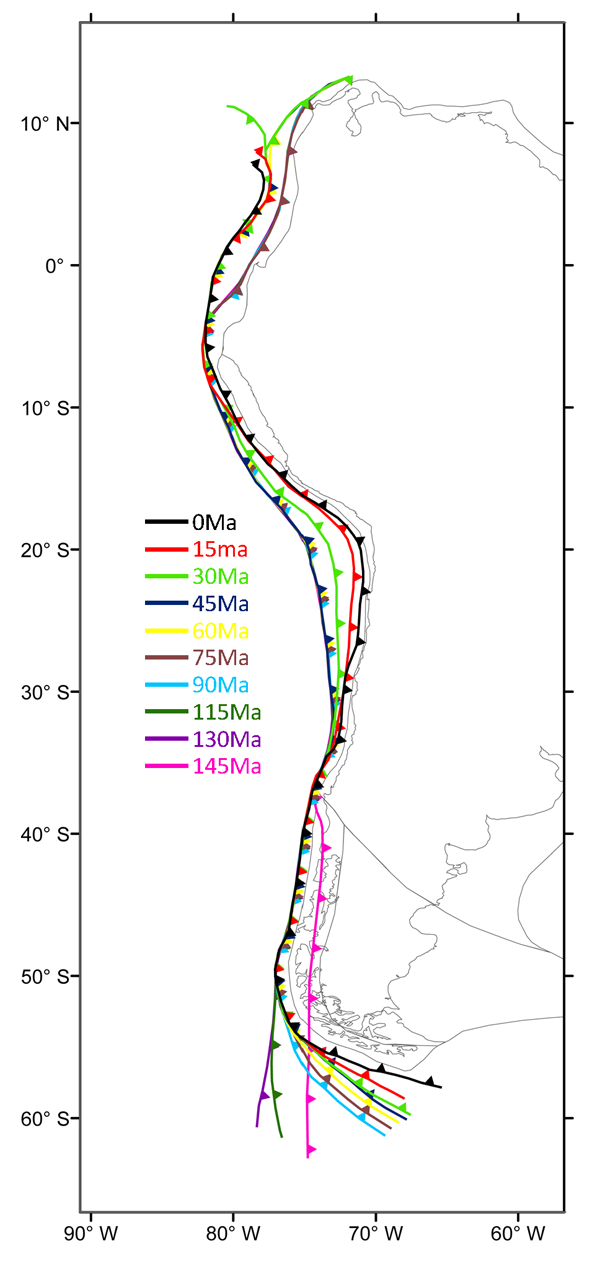
Subduction zone location for distinct times is indicated by coloured lines, with numbers indicating time in Ma. Present-day continental outline is used to illustrate South America.
Strain, dynamic topography, and deformation
The mesh-based feature of Pplates allows the calculation of strain through time. The starting configuration (in this case 145 Ma) of the meshes have zero-strain, and thus the strain reconstruction figures (Figure 6a-j) is presented illustrating the accumulation of strain from 145 ma to the present-day only. Our model predicts the high degree of strain associated with the formation the Patagonian and Bolivian Oroclines. In the Southern Andes it can be seen that the greatest episode of compression occurred between 130-90 Ma, and not only images the strain accumulation associated with closure of the Rocas Verdes Basin, but also the counter-clockwise rotation and associated shortening in the Tierra del Fuego region. The strain model we present here illustrates the high degree of compression (~275 km) that may have occurred during the Mid-Eocene to Mid-Miocene in the Central Andes, with the greatest level of compression occurring in the region of the oroclinal axis. Such data, although of a continental-scale, is potentially useful when quantifying and comparing the degree of deformation to real-world geological data, and alternative palaeogeographic models. As the level of detail within the input data is increased, so is the usefulness of this modelling feature.
Figure 6a-b. Reconstruction illustrating dynamic strain and dynamic topography – 145-130 Ma.
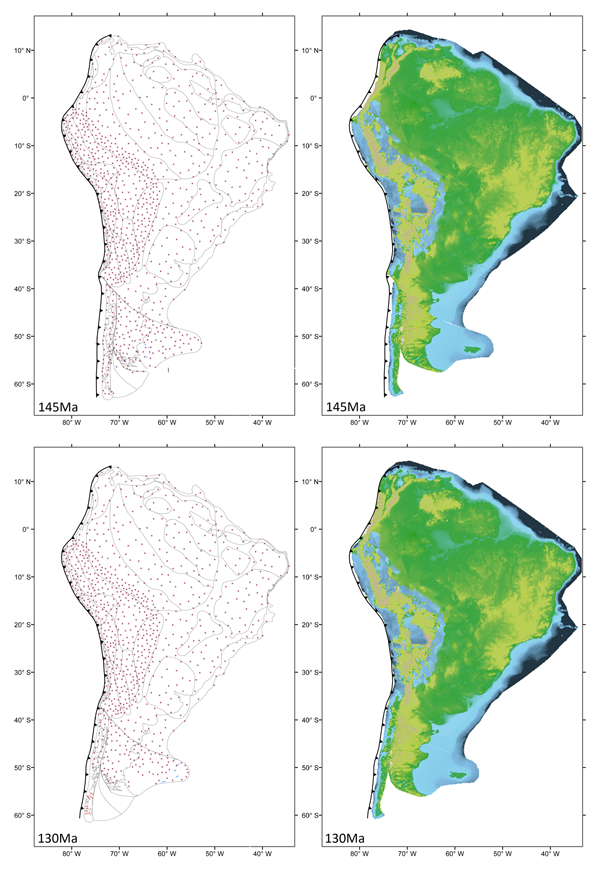
Dynamic strain is illustrated by red lines (compression) and blue lines (extension), while starred points represent no strain. Dynamic topography is depicted by Present-day topography data (NOAA ETOPO2) held by mesh faces. Crustal volume is maintained, although effects of erosion of elevation through time have not been simulated. The model illustrates the presence of basins in the Central Andes prior to the formation of the Bolivian Orocline.
The deforming mesh model of South America also allows changes in topography to be observed (Figure 6a-j). As detailed by Smith et al. (2007), the method for calculating dynamic topography involves the reconstruction starting with present-day topography, assuming isostasy and a standard crustal model, and conserving crustal volume. This calculation illustrates the changes that are forced by uniform stain, as imposed by the reconstruction. While these calculations do not account for erosion, changes in palaeo-altitude caused by crustal thickening are quantified. If the deformations in the model are realistic, such changes in palaeo-altitude could correspond to the depth to basement of the sediment in modern-day basins (Smith et al. 2007).
The dynamic topography model illustrates the potential presence of basins in the Central Andes prior to the formation of the Bolivian Orocline. While this somewhat qualitative information is interesting, the scale of the tectonic reconstruction does not allow genuinely useable conclusions to be drawn regarding basin formation and inversion. Increasing the density of mesh nodes, and incorporating additional geochronological and geological data would go someway to resolving this.