Petrography and mineral chemistry
Herein, the micro-structural features and mineral compositions of three selected samples from the LHS and nine selected samples from the IMS are described in detail, with the aim of correlating their structural and metamorphic evolutions. Relevant microstructures are illustrated in Figs. 6 to 9.
The rock-forming minerals were analysed with a Cambridge Stereoscan 360 SEM equipped with an EDS Energy 200 and a Pentafet detector (Oxford Instruments) at the Department of Earth Sciences, University of Torino. The operating conditions were: 50 seconds counting time and 15 kV accelerating voltage. SEM-EDS quantitative data (spot size = 2μm) were acquired and processed using the Microanalysis Suite Issue 12, INCA Suite version 4.01; natural mineral standards were used to calibrate the raw data; the ΦρZ correction (Pouchou and Pichoir, 1988) was applied. Representative mineral chemistry data are reported in Tables 1-5 and plotted in diagrams of Fig. 10. Links to tables: Table 1, Table 2, Table 3, Table 4, Table 5.
A synthesis of the assemblages-deformation relations is reported in Fig. 11.
Lesser Himalayan Sequence
Sample 09-2
Microstructure
Sample 09-2 is a fine-grained two-micas + chlorite phyllite consisting of mm thick micaceous levels alternated with quartz-rich domains and locally overgrown by porphyroblastic garnet (Fig. 6a, c). In both domains, the main foliation (S2LHS) is defined by the preferred orientation of white mica, biotite and chlorite, in different modal proportions: Wm >> Bt > Chl in the micaceous levels and Bt ≈ Chl >> Wm in the quartz-rich domains. The S2LHS results from the transposition of an older S1LHS foliation still preserved in the microlithons (Fig. 6a, b) and defined by chlorite, white mica and biotite (Chl > Wm > Bt). The S2LHS is locally overgrown by porphyroblastic pink garnet up to several mm in diameter, replaced at the rim and along fractures by a thick corona of chlorite + minor biotite (Fig. 6a). The internal schistosity preserved in garnet porphyroblasts is continuous with the S2LHS and is mainly defined by the alignment of fine-grained ilmenite, quartz and minor epidote. Beside garnet, abundant white mica and biotite lepidoblasts (Fig. 6b, c), hundreds of microns in length, and very rare plagioclase granoblasts and epidote statically overgrow the S2LHS.
A later, mm spaced, planar foliation (S3LHS) nearly orthogonal to the main foliation results from the crenulation of the S2LHS; this S3LHS foliation is only locally evident and is defined by white mica + biotite, as well as by the alignment of ilmenite (Fig. 6d).
Mineral chemistry
The different phyllosilicate generations, clearly recognisable on microstructural basis, are less easily distinguishable on chemical basis being their compositions often overlapped (Fig. 10a, c). White mica shows a Si content in the range 3.06-3.19 a.p.f.u. (on the basis of 11 oxygens); its Na content is inversely proportional to Si and in the range 0.08-0.16 a.p.f.u. On average, white mica in the S1LHS and S2LHS foliations shows higher Si and lower Na contents (Si=3.08-3.19 a.p.f.u.; Na=0.08-0.13 a.p.f.u.) than white mica in the S3LHS and white mica statically overgrowing the S2LHS (Si=3.06-3.14 a.p.f.u.; Na=0.10-0.16 a.p.f.u.). Muscovite flakes statically overgrowing the S2LHS foliation are slightly zoned, with Si decreasing and Na increasing from core to rim. Biotite shows a Ti content in the range 0.10-0.15 a.p.f.u. (on the basis of 11 oxygens) and a slightly variable XMg (XMg=0.32-0.34), inversely proportional to Ti. Biotite in the S1LHS, S2LHS and S3LHS are hardly distinguishable (Ti=0.12-0.15 a.p.f.u.; XMg=0.32-0.33), whereas biotite flakes statically overgrowing the S2LHS have a slightly lower Ti content (Ti=0.10-0.12 a.p.f.u.) counterbalanced by a higher XMg (XMg=0.33-0.34). All the chlorite generations have a Si content in the range 5.07-5.31 a.p.f.u. (on the basis of 36 oxygens) and a XFe=0.64-0.65: therefore, they can be classified as ripidolite according to Hey (1954).
Garnet porphyroblasts are strongly zoned (Fig. 10b): the bell-shaped XMn and XCa decrease from core to rim (XMn from 0.16 to 0.04; XCa from 0.16 to 0.13) is counterbalanced by an increase in both XFe and XMg (XFe from 0.65 to 0.77; XMg from 0.04 to 0.06). The few plagioclase granoblasts overgrowing the S2LHS are oligoclase (Fig. 10d), showing an anorthite content in the range 0.16-0.24, with the lower values toward the rim.
Figure 6. Representative microstructures of LHS samples
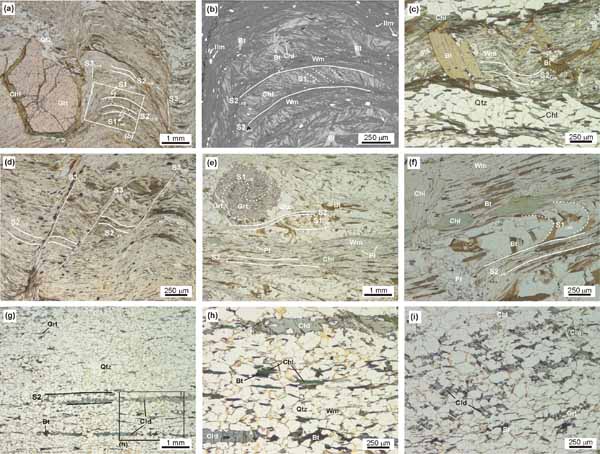
Sample 09-2. (a) Garnet porphyroblast overgrowing the main foliation S2LHS defined by muscovite, biotite and chlorite. The S2LHS is crenulated, with the local development of a later S3LHS foliation, defined by muscovite and biotite. Plane Polarized Light (PPL). (b) Detail of (a), showing microlithons preserving the evidence of an older S1LHS, defined by chlorite, muscovite and biotite. Note the biotite flakes statically overgrowing the S2LHS. Back Scattered Electron image (BSE). (c) Detail of a micaceous level alternated to a quartz-rich domain (note the different modal proportions of phyllosilicates in the two levels). In the micaceous layer, large biotite flakes statically overgrow the S2LHS foliation (PPL). (d) Detail of the S3LHS foliation derived from the crenulation of the main S2LHS foliation (PPL). Sample 09-71a. (e) Snowball garnet porphyroblast mostly enveloped by the main S2LHS foliation, except for its rim GrtR. Garnet core (GrtC) includes a rotated internal foliation (S1LHS) defined by quartz. The S1LHS is also preserved in the microlithons, where it is defined by muscovite and biotite (PPL). (f) Detail of the S2LHS foliation, defined by muscovite and biotite and derived from the transposition of an older S1LHS foliation. Note chlorite and plagioclase porphyroblasts overgrowing the S2LHS (PPL). Sample 09-71b. (g) Microstructure of the chloritoid-bearing quartzite (PPL). (h) Detail of the S2LHS foliation, defined by the alignment of muscovite, biotite, chlorite and chloritoid. The same minerals also overgrow the main foliation (see the top of the microphoto) (PPL). (i) Detail of chloritoid and garnet scheletric crystals (PPL). a and b modified from Mosca et al., 2011.
Sample 09-71a
Microstructure
Sample 09-71a is a phyllitic two-micas schist with snowball garnet porphyroblasts enveloped by a continuous planar foliation defined by mm thick mica-rich layers alternated with quartz-rich domains (Fig. 6e). The main foliation (S2LHS), defined by the preferred orientation of white mica and biotite (Wm > Bt), transposes an older S1LHS foliation preserved in the microlithons (Fig. 6e, f) and defined by both white mica and biotite. The snowball garnet porphyroblasts, several mm in diameter, are mostly enveloped by the S2LHS and contain a rotated internal foliation mainly defined by quartz + minor ilmenite and epidote (Fig. 6e). However, the outermost garnet rims are almost free of inclusions and are in equilibrium with the S2LHS, thus suggesting that garnet growth could have begun synchronously with the development of the S1LHS foliation but ceased at the beginning of the S2LHS development. Quartz, white mica and biotite occur in the pressure shadows of garnet, whereas chlorite and biotite locally replaces garnet at the outermost rim.
Large chlorite lepidoblasts (often in aggregates of radially oriented flakes), plagioclase porphyroblasts and minor biotite and white mica flakes statically overgrow the S2LHS (Fig. 6f).
Mineral chemistry
The three different white mica generations (S1LHS, S2LHS and post-S2LHS) show very similar chemical composition and therefore they are hardly distinguishable on chemical basis (Fig. 10c). Their Si content ranges between 3.07 and 3.16 a.p.f.u. and Na is in the range 0.11-0.21 a.p.f.u. Biotite compositions are more informative (Fig. 11a): biotite in the S1LHS, in fact, shows on average a slightly lower Ti content and a slightly higher XMg (Ti=0.10-0.11 a.p.f.u.; XMg=0.38-0.39) than biotite in the S2LHS and biotite overgrowing the main foliation (Ti=0.10-0.12 a.p.f.u.; XMg=0.37-0.38). Chlorite is homogeneous in composition and it may be classified as a ripidolite (Si=5.15-5.35 a.p.f.u.; XFe=0.56-0.60).
Garnet is zoned and the transition from core to rim is quite sharp (Fig. 10b). Garnet core, corresponding to the snowball central portion of the porphyroblasts, shows a progressive but significant decrease of XMn and XCa (XMn from 0.22 to 0.01; XCa from 0.22 to 0.11) counterbalanced by an increase in XFe and XMg (XFe from 0.55 to 0.80; XMg from 0.02 to 0.08). On the opposite, garnet rim, corresponding to the outermost portion of the porphyroblasts almost free of inclusions, is homogeneous in composition (XMn=0.00; XCa=0.11; XFe=0.80; XMg=0.09). Plagioclase porphyroblasts overgrowing the S2LHS foliation are oligoclase (Fig. 11d), with anorthite content decreasing from core (XCa=0.23) to rim (XCa=0.18).
Sample 09-71b
Microstructure
Sample 09-71b is a chloritoid + garnet -bearing quartzite occurring as a m thick intercalation within the phyllitic two-micas schist 09-71a. The main discontinuous foliation S2LHS is defined by the preferred orientation of white mica, dark brown biotite and very dark green chlorite (Wm > Bt ≈ Chl) (Fig. 6g, h). Chloritoid mainly occurs as scheletric, bluish-green crystals elongated in sub-millimetric levels; also garnet (much more rare) is scheletric and elongated parallel to the S2LHS (Fig. 76i). Chloritoid, chlorite, biotite and white mica also occur in crystals discordant with respect to the main foliation (Fig. 6h, i), probably representing a post-S2LHS assemblage statically overgrowing the S2LHS.
Mineral chemistry
Mineral compositions reflect a relatively Fe-rich bulk rock composition. The dark brown biotite defining the S2LHS foliation shows a Ti content in the range 0.07-0.11 a.p.f.u. and a very low XMg (XMg=0.09-0.13); biotite flakes overgrowing the S2LHS differ for the slightly higher Ti content (Ti=0.11-0.12 a.p.f.u.). White mica in the S2LHS foliation has Si=3.08-3.15 a.p.f.u. and Na=0.03-0.05 a.p.f.u., whereas white mica post-S2LHS shows a lower Si content, in the range 3.02-3.12 a.p.f.u. (Fig. 10c). The two chlorite generations do not show significant differences in composition and may be classified as ripidolite and daphnite (Si=5.00-5.12 a.p.f.u.; XFe=0.88-0.90).
Chloritoid elongated parallel to the S2LHS differs from chloritoid overgrowing the main foliation for its slightly lower XMg (S2LHS: XMg=0.05-0.06; post-S2LHS: XMg=0.06-0.08). Garnet is mainly an almandine (XFe=0.88-0.90), with very little amounts of pyrope, grossular and spessartine components (XMg=0.04-0.05; XCa=0.03; XMn=0.03-0.04) (Fig. 10b).
Inverted Metamorphic Sequence
Sample 09-68
Microstructure
Sample 09-68 is a two-micas augen-gneiss, with albitized K-feldspar porphyroclasts up to few cm in length enveloped by a mylonitic foliation (S2IMS) (Fig. 7a). The S2IMS mylonitic foliation is defined by the preferred orientation of muscovite and biotite (Wm >> Bt), associated to fine-grained quartz and plagioclase and concentrated in millimetric layers alternated to coarse-grained quartzo-feldspathic domains (Fig. 7b). The large K-feldspar porphyroclasts are completely replaced by large albite crystals; rare interstitial grains of microcline have been observed in the quartzo-feldspathic domains (Fig. 7c). A later generation of muscovite and biotite flakes locally overgrows the S2IMS foliation (Fig. 7b). Tourmaline is present as accessory mineral: it is sharply zoned, with a dark green core and a green-yellow rim.
Figure 7. Representative microstructures of the IMS orthogneisses
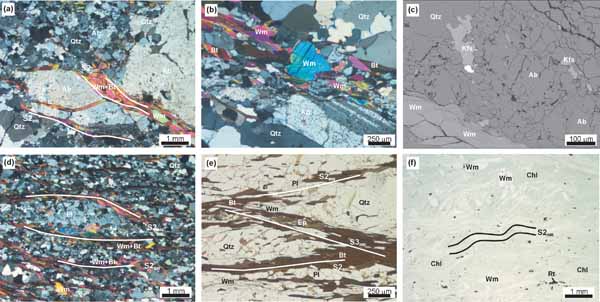
Sample 09-68. (a) Former K-feldspar porphyroclasts (now replaced by albite) enveloped by the main foliation S2IMS, defined by muscovite and biotite. Note the quartz and albite grain reduction along the S2IMS. Crossed Polarized Light (XPL). (b) Detail of the S2IMS foliation overgrown by a muscovite flake (XPL). (c) Detail of interstitial K-feldspar crystals within a fine-grained albite + quartz level (BSE). Sample 09-69. (d) S2IMS mylonitic foliation defined by muscovite and biotite and enveloping a plagioclase lenticular domain derived from the re-crystallization of former K-feldspar. Note the muscovite flakes overgrowing the main foliation (XPL). (e) Detail of a biotite + muscovite + epidote shear band (S3IMS) crosscutting the S2IMS at low angles (PPL). Sample 09-70. (f) Detail of the muscovite + chlorite S2IMS mylonitic foliation crenulated and overgrown by large muscovite flakes (PPL).
Mineral chemistry
The two generations of white mica recognised on microstructural basis (i.e. defining the S2IMS foliation and statically overgrowing the S2IMS) show a very similar composition (Fig. 10c), with a Si content in the range 3.16-3.24 a.p.f.u. and Na=0.04-0.05 a.p.f.u.. The Si content locally decreases down to 3.15 a.p.f.u. at the rim of post-S2IMS lamellae. Biotite defining the S2IMS foliation has Ti=0.11-0.17 a.p.f.u. and XMg in the range 0.41-0.46, inversely proportional to Ti. The post-S2IMS biotite differs for its slightly higher Ti content (Ti=0.17-0.19 a.p.f.u.) (Fig. 10a). Both plagioclase in the quartzo-feldspathic domains and plagioclase replacing K-feldspar porphyroclasts are almost pure albite (An1-3) (Fig. 10d).
Sample 09-69
Microstructure
Sample 09-69 is a two-micas mylonitic orthogneiss with plagioclase-rich lenticular domains enveloped by the main foliation (Fig. 7d). The main, mm spaced, foliation (S2IMS) is defined by the preferred orientation of biotite and muscovite (Bt > Wm) concentrated in sub-mm layers alternated with fine-grained quartzo-feldspathic domains. Very few relics of K-feldspar have been observed in the quartzo-feldspathic domains, although the fine-grained plagioclase-rich elongated domains enveloped by S2IMS foliation derive from the deformation and re-crystallization of former K-feldspar porphyroclasts). A discontinuous foliation (S3IMS) defined by biotite and muscovite crosscuts at low angles the S2IMS foliation (Fig. 7e). Small epidote grains, locally with an allanitic core, overgrow the S2IMS and are associated to the Bt + Wm shear bands.
Mineral chemistry
No significant variations in the white mica composition have been observed as a function of its microstructural position (Fig. 10c). The Si content ranges between 3.11 and 3.20 a.p.f.u., and Na is in the range 0.03-0.05 a.p.f.u. Biotite shows a Ti content inversely proportional to XMg: biotite in the S2IMS foliation and statically overgrowing it has Ti=0.12-0.19 a.p.f.u. and XMg=0.33-0.39, whereas biotite in the later shear bands has a slightly higher Ti content and lower XMg (Ti=0.18-0.20 a.p.f.u.; XMg=0.33-0.34) (Fig. 10a). Plagioclase is an oligoclase (An18-24) (Fig. 10d), with the higher anorthite content corresponding to plagioclase granoblasts overgrowing the S2IMS foliation.
Sample 09-70
Microstructure
Sample 09-70 is a muscovite-chlorite schist occurring as a m thick intercalation within the mylonitic orthogneiss 09-69. The pervasive mylonitic foliation (S2IMS) is defined by the preferred orientation of both white mica and pale-green Mg-rich chlorite, whilst an older foliation (S1IMS) is still preserved in the microlithons and defined by the same assemblage. Abundant large muscovite flakes statically overgrow the S2IMS (Fig. 7f). Apatite and rutile are abundant as accessory phases.
Mineral chemistry
Muscovite and chlorite compositions are quite homogeneous in both the S2IMS and S1IMS. The Si content of muscovite ranges between 3.03 and 3.10 a.p.f.u. (Na=0.17-0.31 a.p.f.u.), and XFe in chlorite is low (XFe=0.28-0.29).
Sample 09-8b
Microstructure
Sample 09-8b is a two-micas, garnet-bearing micaschist consisting of a regular alternation of cm thick muscovite-rich vs. biotite-rich levels, probably reflecting the original juxtaposition of pelitic vs. psammitic layers in the sedimentary protolith. Mineral assemblages and compositions in the two levels are different and will be described separately. The transition from the metapelitic to the metapsammitic level is gradual and occurs through a progressive decrease in the white mica and chlorite modal amounts counterbalanced by an increase in biotite content.
The metapelitic level mainly consists of quartz, muscovite, chlorite, garnet, biotite, staurolite, very minor kyanite and accessory ilmenite and allanite. The main foliation (S2IMS) is defined by the preferred orientation of muscovite, chlorite and biotite (Wm >> Chl > Bt) and envelops very large garnet porphyroclasts up to 1 cm in diameter. Garnet porphyroclasts are microstructurally zoned: garnets core includes an internal foliation (S1IMS) discordant with respect to the S2IMS and defined by quartz and ilmenite, whereas garnets rim is almost free of inclusions and seems in equilibrium with the S2IMS foliation (Fig. 8a). Staurolite occurs as small granoblasts either aligned with the S2IMS foliation or statically overgrowing it (Fig. 8a). Kyanite is very rare and overgrows the main foliation, partially replacing staurolite (Fig. 8d).
Figure 8. Representative microstructures of the IMS metapelitic samples (not-anatectic)
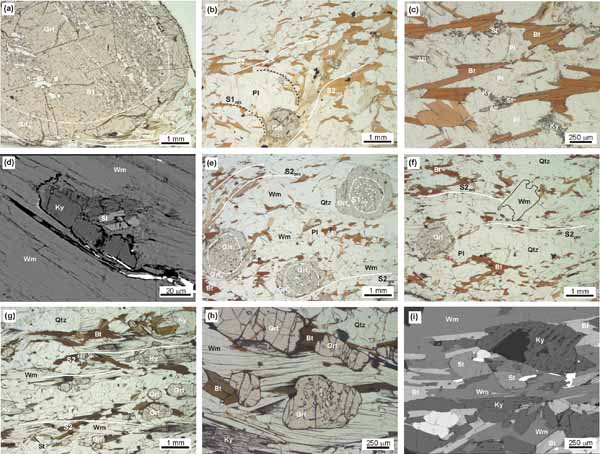
Sample 09-8b. (a) Garnet porphyroblast from a metapelitic level. Garnet core (GrtC) includes an internal foliation (S1IMS) defined by quartz and ilmenite, whereas garnet rim (GrtR) is almost free of inclusions and in equilibrium with the main foliation S2IMS defined by chlorite, muscovite and biotite. Note the staurolite crystal on the right side of the microphoto (PPL). (b) Microstructure of a metapsammitic level: the main foliation, defined by biotite, is overgrown by garnet and plagioclase and derives from the transposition of an older S1IMS foliation. Rutile is present both within and outside garnet (PPL). (c) Detail of a metapsammitic level, showing plagioclase overgrowing the S2IMS. Very fine-grained staurolite and kyanite grow at the interface between adjacent crystals of plagioclase (PPL) (d) Detail from a metapelitic level, showing fine-grained kyanite and staurolite crystals overgrowing the main S2IMS foliation defined by muscovite (BSE). Sample 09-9. (e) Garnet porphyroblasts showing a core (GrtC) crowded of quartz inclusions locally defining an internal foliation (S1IMS), and a rim (GrtR) free of inclusions and in equilibrium with the main foliation (S2IMS). The S2IMS is defined by muscovite and biotite (PPL). (f) Large muscovite flake overgrowing the S2IMS. Note the plagioclase crystal in the same microstructural position, on the right side of garnet (PPL). Sample 09-12. (g) The main foliation S2IMS is defined by the alignment of muscovite, biotite, kyanite and staurolite (PPL). (h) Detail showing garnet rim partially overgrowing the S2IMS (PPL). (i) Detail showing kyanite both aligned with the S2IMS and overgrowing it (PPL).
The metapsammitic level consists of quartz, plagioclase, biotite, garnet, minor muscovite, very minor staurolite and kyanite, and accessory rutile and allanite. The main foliation, parallel to the S2IMS in the adjacent metapelitic level, is defined by the preferred orientation of biotite and muscovite (Bt >> Wm) and is overgrown by garnet and plagioclase porphyroblasts and by a later generation of biotite (Fig. 8b, c). Garnet porphyroblasts include both granoblastic and acicular rutile. Staurolite and kyanite locally occurs at the grain boundaries between adjacent plagioclase porphyroblasts (Fig. 8c), forming very small grains associated with quartz.
Mineral chemistry
Muscovite is the dominant phyllosilicate in the metapelitic level: its Si content ranges between 3.07 and 3.11 a.p.f.u., and Na is in the range 0.15-0.18 a.p.f.u. (Fig. 10c). No significant variations in composition have been observed for the white mica in the metapsammitic level. Biotite prevails in the metapsammitic level and occurs in two different generations (Fig. 10a): biotite in the S2IMS foliation has Ti=0.09-0.12 a.p.f.u. and XMg=0.49-0.52, whereas biotite statically overgrowing the S2IMS has a slightly higher XMg (Ti=0.10-0.11 a.p.f.u.; XMg=0.52-0.53). On average, biotite from the metapelitic level (S2IMS) shows a lower XMg (Ti=0.09-0.13 a.p.f.u.; XMg=0.47-0.51). Chlorite (ripidolite) only occurs in the metapelite level (Si=5.21-5.24 a.p.f.u.; XFe=0.47-0.51).
Garnet porphyroblasts in both levels are strongly zoned but they are significantly different in composition (Fig. 10b). In garnet from the metapelitic level, the XMn and XCa decrease from core to rim (XMn= 0.07 to 0.00; XCa= 0.06 to 0.03), whereas XMg increases, initially slightly and then abruptly passing from 0.07 in the core to 0.18 in the rim. XFe increases from 0.79 in the core to 0.84 in the inner rim, and then decreases down to 0.78 in the outermost rim. Garnet from the metapsammitic level shows a decrease in XMn and XCa from core to rim (XMn=0.05 to 0.01; XCa=0.25 to 0.15) counterbalanced by an increase in both XMg and XFe (XMg=0.11 to 0.16; XFe=0.60 to 0.72).
Plagioclase is present only in the metapsammitic level. It is mostly an andesine (An31-37), with rare oligoclase cores (An18-21) (Fig. 10d). Staurolite mostly occurs in the metapelitic level: its XMg varies from XMg=0.15-0.18 for the staurolite in equilibrium with the S2IMS foliation to XMg=0.14-0.16 for the staurolite overgrowing the S2IMS. The fine-grained staurolite in the metapsammitic level has XMg=0.14-0.16.
Sample 09-9
Microstructure
Sample 09-9 is a coarse-grained two-micas, garnet-bearing micaschist. The main foliation (S2IMS) is defined by the preferred orientation of muscovite and biotite (Wm ≈ Bt) occurring in mm thick micaceous levels alternated to quarzo-feldspathic domains (i.e. Qtz + Pl) (Fig. 8e, f). Large garnet porphyroblasts (up to several mm in diameter) are partially enveloped by the main foliation. More in detail, garnet porphyroblasts are microstructurally zoned: garnet core is crowded of inclusions (quartz, rutile and very fine-grained graphite) locally defining a rotated internal foliation (S1IMS), whereas garnet rim is almost free of inclusions (Fig. 8e). Garnet rim is often discontinuous and appears in equilibrium with the S2IMS foliation. Large muscovite flakes and less frequent biotite lamellae statically overgrow the main foliation (Fig. 8f). Rutile is rimmed by ilmenite in the matrix.
Mineral chemistry
Except for the zoned garnet porphyroblasts, mineral compositions are extremely homogeneous thus reflecting the attainment of equilibrium. Biotite shows a Ti content in the range 0.18-0.21 a.p.f.u. and XMg=0.36-0.38 (Fig. 10a). White mica has Si=3.09-3.17 a.p.f.u. and low Na content (Na=0.07-0.09 a.p.f.u.) (Fig. 10c): no significant differences have been observed between muscovite in the S2IMS and muscovite post-S2IMS. Plagioclase is oligoclase, with An=20-25 (Fig. 10d). Garnet is zoned, with XMn and XCa decreasing (XMn=0.07 to 0.02; XCa=0.13 to 0.11) and XMg and XFe increasing (XMg=0.07-0.11; XFe=0.72-0.75) from core to rim (Fig. 10b).
Sample 09-11
Microstructure
Sample 09-11 is a garnet-bearing, two-micas gneiss with a main foliation (Sm) defined by discontinuous micaceous levels (Bt > Wm) alternated with dominant quarzo-feldspathic layers (Fig. 9a). In the quartzo-feldspathic domains most of quartz and plagioclase are coarse-grained, whereas K-feldspar occurs as interstitial crystals, often with cuspate shapes (i.e. “melt pseudomorphs” according to the definition of Holness and Clemens, 1999; Holness and Sawyer, 2008). In those domains where K-feldspar is abundant, muscovite is absent. Plagioclase often presents a spongy rim due to the presence of very fine-grained K-feldspar exsolutions.
Figure 9. Representative microstructures of the structurally upper IMS anatectic metapelitic samples and of lower HHC “Barun-type” gneiss
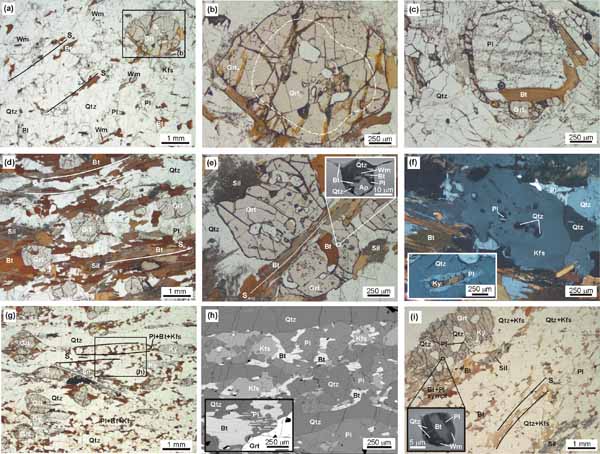
Sample 09-11. (a) The gneissic foliation Sm is defined by the alignment of both muscovite and biotite (PPL). (b) Detail of garnet porphyroblast shown in (a). Garnet core (GrtC) includes coarse grained rounded quartz, whereas garnet rim (GrtR) is crowded of very small polymineralic inclusions with a negative crystal shape (i.e. nanogranites) (PPL). (c) Detail of coronitic garnet isolating plagioclase and biotite from quartz. Note that coronitic garnet includes nanogranites as the garnet rim in (b) (PPL). Sample 09-13a. (d) The main foliation Sm is defined by the alignment of biotite, partially replaced by fibrolitic sillimanite, and overgrown by garnet porphyroblasts (PPL). (e) Detail of a garnet porphyroblast crosscut by a shear band defined by biotite + sillimanite (Sm+1). Garnet includes both coarse grained biotite and quartz and very small polymineralic inclusions (i.e. nanogranites) (PPL). One of these nanogranites, consisting of quartz, biotite, muscovite and albitic plagioclase is reported in the inset (BSE). (f) Detail of a coarse grained K-feldspar crystal with rounded inclusions of plagioclase and quartz, partially overgrowing the Sm (XPL). The inset shows a kyanite relict separated from quartz by a continuous corona of plagioclase (XPL). Sample 09-14. (g) The main foliation Sm is defined by the alternation of plagioclase + biotite + K-feldspar layers with elongated quartz rods. Garnet and kyanite are enveloped by the plagioclase + biotite + K-feldspar layers. The Sm is crosscut by later shear bands (Sm+1) defined by biotite + sillimanite (PPL). (h) Detail of the plagioclase + biotite + K-feldspar levels shown in (g) (BSE). The inset shows a detail of the biotite + plagioclase symplectitic microstructure developed at the interface between biotite and garnet (BSE). Sample 09-16a. (i) The gneissic foliation SHHC is defined by biotite + sillimanite + plagioclase discontinuous mesocratic levels alternated with quartzo-feldspathic leucocratic layers (PPL). Garnet porphyroblast shows two types of inclusions: coarse-grained rounded quartz and kyanite surrounded by a thin film of plagioclase (“melt pseudomorph”) and “nanogranite” inclusions consisting of biotite, white mica, quartz and albitic plagioclase. One of these nanogranites is shown in the inset (BSE).
Garnet occurs in two different microstructural positions: (i) as mm large porphyroblasts set in the quartzo-feldspatic domains (Fig. 9b); (ii) as thin and discontinuous corona around plagioclase and/or biotite (Fig. 9c). The core of garnet porphyroblasts includes large and rounded inclusions of quartz, whereas the outermost rim is crowded of very small (tens of microns) birefringent inclusions which are too small to be deciphered at the optical microscope (Fig. 9b). At the SEM, these inclusions show a negative crystal shape and are polymineralic, generally consisting of quartz + muscovite ± biotite ± chlorite ± rutile. The same micrometric polymineralic inclusions are present in the garnet coronas developed at the interface between plagioclase and quartz or biotite and quartz. These inclusions are interpreted as “nanogranites” according to the definition of Cesare et al. (2009) and demonstrate that the rim of porphyroblastic garnet and coronitic garnet grew in the presence of melt, i.e. they are peritectic phases likely grown during de-hydration melting of muscovite (Vielzeuf and Holloway, 1988, Tajcmanova et al., 2011).
Mineral chemistry
Biotite and muscovite have very homogeneous compositions (Fig. 10a, c): Ti content in biotite is in the range 0.16-0.21 a.p.f.u. and its XMg is 0.29-0.30, whereas the Si content in muscovite is 3.11-3.12 a.p.f.u. The microstructural zoning of plagioclase corresponds also to a chemical zoning: both plagioclase core and rim are oligoclase in composition (Fig. 10d), but the anorthite content of the core is lower than that of the rim (core: An11-17, Or2-3; rim: An15-20, Or0-2).
Figure 10. Compositional diagrams for biotite (a), garnet (b), white mica (c) and plagioclase (d) in the studied samples
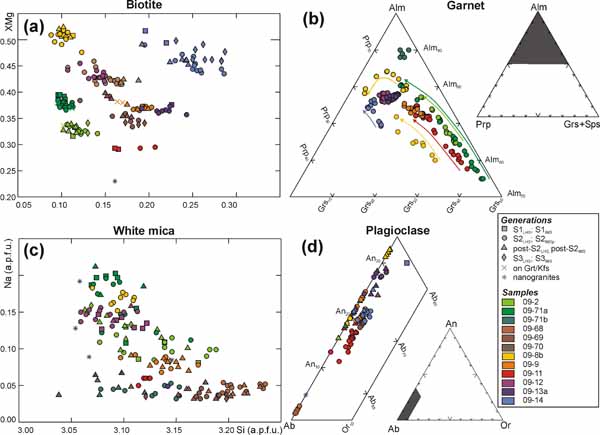
For each sample, biotite, muscovite and plagioclase have been distinguished according to their microstructural position. Garnet zoning from core to rim is indicated in (b) with coloured arrows; dotted vs. continuous arrows for sample 09-8b refer to garnet from meta-psammitic vs. metapelitic levels, respectively.
Garnet porphyroblasts are strongly zoned (Fig. 10b). Garnet cores show a bell-shaped decrease of XMn from 0.15 in the inner core to 0.03 in the outer core, counterbalanced by an increase in both XMg and XFe (XMg=0.04 to 0.09; XFe=0.58 to 0.72). The XCa trend is more complex, increasing from 0.22 to 0.24 and then decreasing down to 0.15. In the garnet rims crowded of ”nanogranites”, XMn and XFe increase (XMn=0.04 to 0.15; XFe=0.68 to 0.75) and XMg and XCa decrease (XMg=0.09 to 0.07; XCa=0.12 to 0.07). Coronitic garnet around plagioclase and/or biotite shows the same composition as the rim of porphyroblastic garnet, with the highest XMn and lowest XMg values toward the rim.
Sample 09-12
Microstructure
Sample 09-12 is a coarse-grained two-micas + garnet + staurolite + kyanite schist. The main foliation (S2IMS) is defined by the preferred orientation of muscovite and biotite (Wm > Bt), which are concentrated in mm thick continuous layers alternated to quartz-rich domains (Fig. 8g). Locally, large muscovite flakes resembling mica-fish suggest that the main foliation S2IMS derived from the transposition of an older S1IMS foliation. Garnet occurs as mm large crystals which appear in equilibrium with the main foliation (Fig. 8g), except for the outermost rim that locally overgrows the S2IMS (Fig. 8h). Small quartz inclusions are locally present within garnet. Staurolite and kyanite occur as coarse-grained crystals either aligned with the S2IMS or overgrowing it (Fig. 8i). Neither staurolite nor kyanite are included in garnet; if in contact with garnet, they appear in equilibrium with it. Large muscovite flakes statically overgrow the main foliation.
Mineral chemistry
White mica occurs in three different generations (Fig. 10c): the large muscovite flakes enveloped by the main foliation (i.e. S1IMS) show a Si content in the range 3.08-3.13 a.p.f.u. and Na=0.12-0.15 a.p.f.u.; muscovite defining the S2IMS shows a lower Si (Si=3.06-3.11 a.p.f.u.) counterbalanced by a slightly higher Na content (Na=0.14-0.16 a.p.f.u.); finally, the large muscovite flakes overgrowing the S2IMS have Si=3.09-3.11 a.p.f.u. and Na=0.14-0.16 a.p.f.u.. Biotite is quite homogeneous in composition (Fig. 10a) with Ti=0.11-0.15 a.p.f.u. and XMg=0.42-0.45.
Garnets are almost homogeneous (Fig. 10b), with XMg=0.15, XCa=0.04, XMn=0.05 and XFe=0.76: a slight decrease in XMg (down to 0.13), counterbalanced by an increase in XMn, is observed at the outermost rim. Staurolite is also homogeneous, with XMg ranging from 0.14 to 0.16.
Sample 09-13a
Microstructure
Sample 09-13a is a anatectic garnet + biotite + sillimanite gneissic micaschist, consisting of quartz, K-feldspar, plagioclase, biotite, sillimanite, and minor kyanite and muscovite. The main foliation (Sm) is defined by layers of biotite + sillimanite alternated with quartzo-feldspathic domains (Fig. 9d). Two types of quartzo-feldspathic domains may be recognised: (i) coarse-grained discontinuous levels mainly consisting of quartz (including very rare muscovite flakes) partially overgrown by fibrolitic sillimanite, and (ii) finer-grained K-feldspar + quartz + plagioclase layers. K-feldspar generally includes rounded quartz and/or plagioclase grains (i.e. peritectic K-feldspar: Fig. 9f) and it is partially corroded at its rim by plagioclase. Biotite occurs in two different microstructural positions: (i) it defines the Sm, where it appears partially replaced by fibrolitic sillimanite and overgrown by plagioclase granoblasts (Fig. 9d), and (ii) it defines mm thick shear bands (Fig. 9e), intersecting the Sm at low angles, in equilibrium with fibrolitic sillimanite.
Mm large crystals of garnet overgrow the main foliation Sm. Garnet is crowded of two different types of inclusions (Fig. 9e): (i) large mono- and/or polyminineralic inclusions mainly consisting of rounded crystals of quartz and biotite; (ii) very small (tens of microns) polymineralic inclusions with negative crystal shape, consisting of quartz, biotite, white mica and albitic plagioclase in different proportions and generally including rutile or apatite crystals (i.e. “nanogranites” according to the definition of Cesare et al., 2009) (Fig. 9e). These inclusions suggest that garnet is a peritectic phase grown during de-hydration melting of biotite. Garnet crystals are locally crosscut by the biotite + sillimanite shear bands and they are partially corroded at their rim by fibrolitic sillimanite (Fig. 9e). Rare relict kyanite crystals, always rimmed by a plagioclase corona, also occur in the quartzo-feldspatic domains (Fig. 9f). Finally, rare muscovite flakes locally overgrow the main foliation.
Mineral chemistry
Biotite composition is quite homogeneous (Fig. 10a), independently from its microstructural position: Ti content is in the range 0.19-0.24 a.p.f.u. and XMg=0.36-0.38. Biotite in the very small polymineralic inclusions within garnet has a significant lower Ti and XMg contents (Ti=0.16 a.p.f.u.; XMg=0.23). Plagioclase is mainly an oligoclase (An21-29): on average, the lowest anorthite contents (An21-26) are observed in plagioclase overgrowing the Sm biotite (Fig. 10d). Plagioclase in the very “nanogranite” inclusions within garnet is albite (An2-5).
Garnet is almost unzoned (Fig. 10b), with XMg=0.13-0.14, XCa=0.04-0.05, XMn=0.05-0.06 and XFe=0.86-0.87; XMg slightly decreases toward the rim, down to XMg=0.11, counterbalanced by an increase in XFe. The rare muscovite flakes included in coarse-grained quartz have Si=3.14 a.p.f.u. and Na=0.05 a.p.f.u., whereas the late muscovite flakes statically overgrowing the Sm show lower Si (Si=3.07-3.10 a.p.f.u.) and higher Na (Na=0.06-0.07 a.p.f.u.) contents.
Sample 09-14
Microstructure
Sample 09-14 is a anatectic garnet + biotite + kyanite banded gneiss. The main foliation (Sm) is defined by mm thick continuous mesocratic layers consisting of biotite + plagioclase + K-feldspar alternated with leucocratic quartz domains, in which quartz mainly occurs as coarse-grained rods (Fig. 9g, h). Biotite in the mesocratic layers does not show any preferred orientation. Mm large garnet and kyanite crystals are partially enveloped by the mesocratic layers (Fig. 19g, h). Garnet mainly includes rounded quartz and rutile, especially in the core, and more rare biotite and plagioclase and is partially corroded by kyanite and biotite at its rim. Peculiar symplectitic microstructures consisting of biotite + plagioclase often occur at the contact between garnet and biotite (Fig. 9h). These symplectitic microstructures are generally interpreted, in anatectic rocks, as related to the late interactions between solids and melt (i.e. back-reactions), during final melt crystallization (e.g. Waters, 2001; Cenki et al., 2002; Kriegsman and Álvarez-Valero, 2010). Mm thick and cm spaced shear bands, defined by biotite, sillimanite and plagioclase, crosscut the Sm at low angles (Fig. 9g).
Mineral chemistry
Biotite shows significant different compositions as a function of its microstructural position (Fig. 10a). Ti content is inversely proportional to XMg, varying in the range: Ti=0.20-0.23 a.p.f.u. (XMg=0.49-0.52) for biotite included in garnet, Ti=0.24-0.30 a.p.f.u. (XMg=0.43-0.47) for biotite in the mesocratic domains, Ti=0.20-0.26 a.p.f.u. (XMg=0.45-0.49) for biotite in the Bt + Pl symplectites and Ti=0.24-0.30 a.p.f.u. (XMg=0.46-0.50) for biotite in the shear bands. Plagioclase is oligoclase in composition (An19-25) and no significant compositional variations have been observed in different microstructural sites (Fig. 10d). Garnet is almost unzoned (Fig. 10b), with homogeneous XMg=0.19 and XMn=0.02, and a very slight decrease of XCa from 0.10 in the core to 0.05 in the rim, counterbalanced by a slight increase in XFe (XFe=0.80-0.83).
Higher Himalayan Cristallines
Sample 09-16a
Microstructure
Sample 09-16a is a biotite + garnet + K-feldspar + sillimanite + kyanite gneiss. The main foliation (SHHC) is defined by thin discontinuous and mesocratic layers consisting of biotite, sillimanite ± fine-grained plagioclase alternated with centimetric leucocratic quartzo-feldspathic levels consisting of quartz and K-feldspar and minor coarse-grained anti-perthitic plagioclase (Fig. 9i). In the leucocratic domains, cuspate shaped domains of quartz and plagioclase are locally abundant in contact with rounded quartz, plagioclase and especially biotite: these microstructures are interpreted as “melt pseudomorphs” (Holness and Clemens, 1999; Holness and Sawyer, 2008).
Large garnet porphyroblasts (up to 1 cm in diameter) are present in the leucocratic layers (Fig. 9i). Two different types of inclusions have been observed within garnet porphyroblasts: (i) coarse grained mono- or poly-mineralic inclusions, mainly consisting of rounded quartz and/or corroded biotite and kyanite locally surrounded by a thin film of plagioclase, with garnet showing crystal faces toward plagioclase; (ii) very small birefringent inclusions (up to 20 microns in diameter) with a negative-crystal shape, mainly consisting of quartz, biotite, K-feldspar and albitic plagioclase in different proportions (i.e. “nanogranites” according to Cesare et al., 2009). The two types of inclusions clearly suggest that garnet is a peritectic phase, grown in the presence of melt during de-hydration melting of biotite, at the expenses of quartz, biotite and kyanite. Garnet porphyroblasts are partially replaced at their rim by biotite + plagioclase symplectitic microstructures, interpreted as the result of back-reactions between solid and melt during final crystallization of the melt (e.g. Kriegsman and Àlvarez-Valero, 2010). Kyanite is rare and mainly included in garnet, whereas sillimanite is only present in the mesocratic discontinuous biotite + plagioclase layers defining the SHHC.
Mineral assemblage and microstructural features of sample 09-16 are perfectly comparable with those described by Groppo et al. (2012) for the lateral equivalents Barun Gneisses of the Everest-Makalu region. The mineral chemistry and P-T evolution of these anatectic metapelitic gneisses has been already presented and modelled by Groppo et al. (2012), therefore they will not be further discussed in this paper.