The Rhodope Metamorphic Complex: A part of the Alpine orogenic system
Historical perspective
A rosy-cheeked (etymology of Rhodope) Naiad nymph, queen of Thrace, and her husband, king Haimos, were transfigured into mountains after they dared compare themselves to, and so offend the celestial and almighty couple Hera and Zeus. Yet, Zeus showed some indulgence since he did not separate them: Haimos was metamorphosed into the neighbouring Balkan Mountains (Ovid, Metamorphoses, book 6. 87-89). The mythology narrates that Rhodope was the daughter of the river-god Hebros, hence the grand-daughter of Okeanos and his sister and wife, Tethys.
Much geological work since the late 1980’ has re-established the affiliation between Rhodope and Tethys, the long-recognized oceanic basin from which the Alpine-Himalayan orogenic system rose [Suess, 1888].
The oldest description of gneissic and plutonic rocks in the Rhodope is due to Boué [1836] and the name "Massif de Rhodope" to Viquesnel [1853]. The belief of the time was that such rocks should be old, such that crystalline rocks of the Balkan and Rhodope Mountains were considered as pre-Alpine basement
Figure 2. Sketch map of the Rhodope Metamorphic Complex.
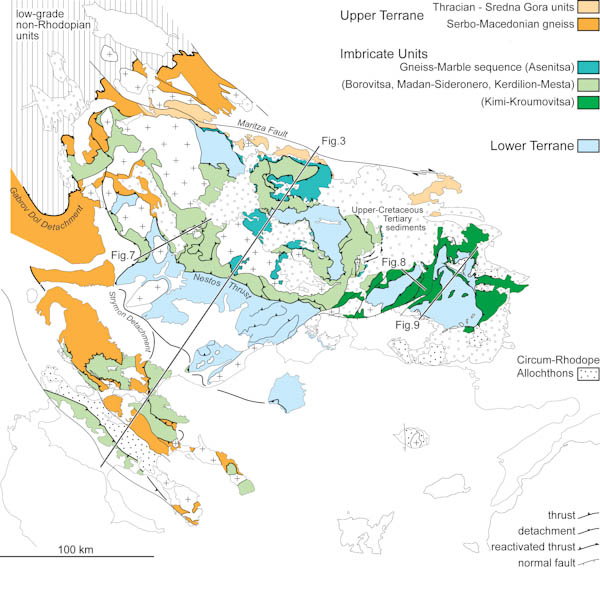
Denudation of the northern part, along the Gabrov Dol Detachment, is older than the crosscutting, Turonian (ca 75 Ma) pluton (Table 7 and Fig. 10). The Strymon Detachment is the larger identified Cenozoic denudation fault. Several Rhodope thrust sheets have been further displaced during extensional tectonics and coeval formation of the Cenozoic basins.
[Cvijič, 1904]. Some authors compared these gneisses to those of the eastern Alps and suggested that they "received" their tectonic character from the "movements" that formed the whole Alpine fold belt [Kossmat, 1924]. This interpretation was possibly too daring for the time and Kober [1921; 1928; 1929] suggested that the Rhodope was a rigid "Zwischengebirge" between two branches of the Alpine chains: the Dinarides-Hellenides on the one side, and the Carpathians-Balkanides on the other. Kober considered the Vardar ophiolitic zone [his Narbe root zone; Kober, 1952]) as the major boundary between the two Alpine branches and attributed the Rhodope to the South-Balkan realm, extending from the Vardar Suture zone northeastwards. Since then, the Rhodope as a geological entity has been placed between the Vardar (Axios in Greece) valley to the west, the Aegean Sea to the south, and the Maritza Fault to the north (Figs. 1 and 2). The old-basement interpretation was reiterated for some years [Jaranoff, 1938; Vergilov et al., 1963; Boncev, 1971; Foose and Manheim, 1975; Pal'shin et al., 1975; Kozhoukharov et al., 1988] and accepted for early plate tectonics descriptions [Dewey et al., 1973; Hsü et al., 1977; Burchfiel, 1980], despite clear statements on Alpine tectonics and metamorphism by few authors who followed Kossmatt's ideas [Petraschek, 1931; Janichevsky, 1937; Gâlâbov, 1938]. "Dinaride"-type orogen (i.e. southwestward thrusting of Alpine orogeny) was even emphasised for the eastern Rhodope [Jaranoff, 1938]. Admittedly, such emphasis was mostly based on feelings rather than on data, and the same authors accepted that most Rhodopean granites and gneisses were Precambrian or Variscan. A stable Rhodope continental block during the Alpine orogeny was questioned in Greece [Meyer, 1968] and Bulgaria [Ivanov, 1988]. Its ancient origin was brought into dispute from:
- (1) the lack of Palaeozoic and/or Mesozoic sedimentary cover as already noted by Viquesnel [1853] but the point was forgotten by those who worked after him, although this cover is typical of basement regions in southern Europe, even in the Strandja Massif, next to and northeast of the Rhodope Metamorphic Complex [e.g. Görür et al., 1997];
- (2) early geochronological determinations pointing to Mesozoic [Zagorčev and Moorbath, 1983; 1986; Soldatos et al., 2008] to Eocene-Oligocene [Borsi et al., 1965; Meyer, 1968; Pal'shin et al., 1975] protolith ages of gneiss and "synkinematic" granitoids;
- (3) the consistency of synmetamorphic structures and kinematics with the bulk Mesozoic convergence between Europe and Africa and
- (4) a still thick crust with the Moho lying at ca. 50 km depth [Dačev and Petkov, 1978; Geiss, 1987].
The alternative was that the Rhodope is a complex of Alpine synmetamorphic nappes formed during closure of the Tethys [Burg et al., 1990; Koukouvelas and Doutsos, 1990]. Multiphase recumbent folding had been established by several structural studies of the Rhodope gneissic complex, both in Bulgaria [Ivanov et al., 1984; Ivanov et al., 1985] and in Greece [Papanikolaou and Panagopoulos, 1981]. Polyphase deformation was a hint as to the correctness of the present-day structural interpretations whereby early thrusting and thickening of the crust has been largely overprinted by extensional metamorphic core complexes and associated low-angle detachment faults [Kolocotroni and Dixon, 1991; Dinter and Royden, 1993; Sokoutis et al., 1993; Brun and Sokoutis, 2007]. Ductile extension makes a link with brittle extension that controlled formation of the Cenozoic sedimentary basins widely distributed over the Rhodope [e.g. Tzankov et al., 1996]. The Rhodope nappe stack was overlain transgressively by Lutetian/ Priabonian (48–42 Ma) deep- to shallow-water sediments [Krohe and Mposkos, 2002] and was intruded by large-scale Tertiary granitoids, which led to local migmatisation of the host rocks [e.g. Peytcheva et al., 2004; Liati, 2005].
Boundaries of the Rhodope
Kossmat [1924] first proposed that the Vardar Zone separates the Rhodope Massif, to the east from the Pelagonian Massif, to the west. He extended the Rhodope, through the Aegean Sea, to Anatolia (Asia Minor).
The Serbo-Macedonian Massif was separated from the Rhodope Massif by Kockel and Walther [1965] based on the observation of different metamorphic grades on either side of the Strymon Valley and apparent differences in lithological contents and inferred ages. Kockel and Walther [1965] placed the boundary on the eastern border of the Tertiary Strymon Basin along their west-dipping Strymon Thrust, later revealed to be a Miocene normal fault [Dinter and Royden, 1993]. Similar lithologies with similar protolith and Cretaceous metamorphic ages on both sides of the Srymon basin [Himmerkus et al. 2007 and 2009a] show that distinction between the Serbo-Macedonian and the Rhodope is not necessary.
As envisaged nowadays, the Rhodope thrust system incorporates the Serbo-Macedonian Massif such that the Rhodope Massif extends to where early authors placed its western boundary: along the Vardar Suture Zone [Ricou et al., 1998]. The northern boundary of the Rhodope Massif is the dextral Maritza strike-slip fault (Fig. 2), which deforms late Jurassic granitoids [Naydenov et al., 2009] and developed Late Cretaceous (ca. 100 Ma, 40Ar/39Ar) syn-metamorphic shear fabrics [Velichkova et al., 2004; Rieser et al., 2008].
To the northwest, in Bulgaria, the top-to-NW Gabrov Dol Detachment (Fig. 2) separates amphibolite-facies rocks now attributed to the Rhodope Massif, from lower grade sequences (Struma Diorite, Vlasina and Frolosh formations in the literature) and non- or weakly-metamorphosed and fossiliferous Palaeozoic sequences unconformably overlain by Permian detrital sediments [Bonev et al., 1995]. This detachment is older than the crosscutting 73 Ma Plana pluton [Boyadjiev, 1981]. Although this K-Ar age constraint needs confirmation from a more robust isotopic system, it is supported by the 82.25 ± 0.22 Ma U/Pb zircon age of the Varshilo Granite, which belongs to the same plutonic system as the Plana Granite [Von Quadt and Peytcheva, 2005], and by the ca. 90 Ma zircon fission-track ages in both the footwall and hanging wall of the detachment [Kounov et al., 2010]. Importantly, these ages demonstrate that at least the northwestern Rhodope was already tectonically unroofed by Late Cretaceous times. To the east, gneiss with similar characteristics as those reviewed below might be a lateral extension of the Rhodope Metamrophic Complex, in Turkey [Bonev and Beccaletto, 2007].
General structure, strain and kinematics
The overall structure of the Rhodope is the 300 x 300 km, open antiform identified in early work (Fig. 3). This NW-SE antiform bends lithological contacts transposed into the regional main-phase foliation during multiphase, tight to isoclinal recumbent folding. Multiphase designates a sequence of structures overprinting older structures that pertain to several generations of mostly coaxial folds [e.g. Meyer, 1969; Papanikolaou and Panagopoulos, 1981; Ivanov et al., 1985; Burg et al., 1996b]. Strain gradients from protomylonites to ultramylonites and ubiquitous sense-of-shear criteria indicate that foliation and folds result from intense, non-coaxial ductile deformation [Burg et al., 1990; Burg et al., 1993]. Ductile shear zones were active under amphibolite-facies conditions and delineate tectonic contacts between terranes with distinct structural-metamorphic histories. Therefore, the Rhodope metamorphic complex is viewed as a region of large-scale nappe tectonics (Figs. 2 and 3).
Figure 3. Rhodope Metamorphic Complex.
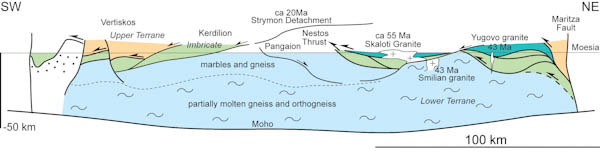
Synthetic cross section of the Rhodope Metamorphic Complex (approximate trace on Figure 2). Moho depths after Velchev et al. [1971], Dačev and Petkov [1978] and Geiss [1987].
The bulk structure is simplified as a crustal-scale, synmetamorphic, amphibolite-facies duplex [Ricou et al., 1998]: the top and bottom units are different associations of paragneiss, orthogneiss and marbles. They are the hanging wall and footwall of a complex imbrication including meta-ophiolites and relicts of a Jurassic magmatic arc. These imbricates, previously lumped under the term of intermediate units [Ricou et al., 1998], define a dismembered suture. Thrusts placed higher grade rocks onto lower grade rocks during intermediate- to high-pressure metamorphic conditions, before pervasive, syn-kinematic equilibration in amphibolite- and greenschist-facies conditions [Burg et al., 1996a; Burg et al., 1996b]. The main-phase foliation and shear zones contain the dominantly, almost homogeneous north-northeast lineation pattern defined by isoclinal and exceptionally sheath-fold axes, mineral lineations, and boudinage [Burg et al., 1996a; Burg et al., 1996b]. The general attitude of mylonites, foliations and stretching lineations (Fig. 4) demonstrate a regionally consistent, bulk southwestward thrusting [Burg et al., 1990; Kilias and Mountrakis, 1990; Burg et al., 1996a; Barr et al., 1999]. Finite strain measurements and quartz fabrics indicate that deformation was close to plane strain [Burg et al., 1996b]. In Greece, the intermediate and lower units were identified as Upper and Lower Units [Papanikolaou and Panagopoulos, 1981], respectively. At variance with earlier descriptions, and in the light of more recent work referred to hereafter, the hanging-wall terrane of this review is continental (the Serbo-Macedonian of previous authors), while the ophiolitic unit defined as roof unit in Burg et al. [1993] is now considered as one of the intermediate imbricates.
Erosion, tectonic denudation and deposition of colluvial - proluvial sediments unconformable on the metamorphic rocks occurred as early as Maastrichtian - Paleocene times [Boyanov et al., 1982; Goranov and Atanasov, 1992]. This was followed by a period of apparent quiescence (a question that needs clarification) before widespread, graben-forming extension and intermediate to acid volcanism in Eocene-Oligocene times. Some thrust zones were then occasionally reworked as low-angle normal faults. The structural overprint leads to disputes where folded thrusts are tilted to attitudes with apparent normal sense of shear. Yet, there are distinct, low-dip shear zones and brittle normal faults that additionally displaced parts of the thrust system [Burg et al., 1990; Krohe and Mposkos, 2002].
The amount of Cenozoic volcanism above a subduction zone, the unusual high topography and large crustal thickness today hint at possible similarities between the Andes and the Rhodope mountains that have been discussed, up to now, in terms of collision only. This question refers to first-order features that will be examined in the interpretation paragraph of this review.
A second phase of general extension began in the late Miocene. It is still active and related to the Aegean extension in the hanging wall plate of the rolling back Aegean subduction [e.g. Zagorčev, 1992].
Figure 4. Map of stretching lineations over the Rhodope metamorphic Complex.
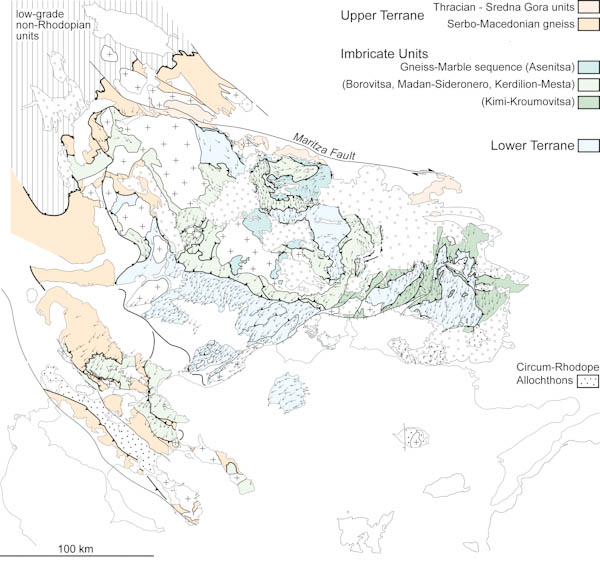
All arrows point towards the local shear direction. Note that the pattern does not allow separating lineations of different age clusters. Same symbols as Figure 2.
Plate tectonic setting - geophysical constraints
More than 2500 km of anticlockwise, rotational convergence between Africa and Europe since about 140 Ma has produced the Hellenides-Dinarides-Rhodope orogenic system [Savostin et al., 1986; Schettino and Scotese, 2005]. Paleogeographic reconstructions agree on the implication of several continental blocks derived from Gondwana and separated by Tethys-related oceanic basins [Ricou et al., 1998; Stampfli and Borel, 2002]. Seismic tomography images a continuous, slab-type high-velocity anomaly to about 1500 km depth, with a 300 km flat segment lying on the 660 km discontinuity [Bijwaard et al., 1998]. The length of the slab fits the inferred amount of post-Jurassic convergence, whilst the 300 km long flat slab would measure the amount of slab rollback responsible for the late Cenozoic extension in the Aegean [Van Hinsbergen et al., 2005]. These results imply that no slab breakoff has occurred on the Vardar-Aegean side since the Jurassic. They also suggest that the Mediterranean slab did not begin to subduct under the Aegean continental plate in Miocene times but instead was continuously subducted at an average convergence rate of 2-2.5 cm/a over the last 100 Ma [Hafkenscheid et al., 2006]. Subduction possibly involved several continental and oceanic lithospheres [e.g. Jolivet and Brun, 2010].
Paleomagnetic measurements document a clockwise rotation of much of the Rhodope by > 12° since the mid-Oligocene [Dimitriadis et al., 1998]. Therefore, it is almost due to unfortunate coincidence that post-Oligocene, extension-related, NE-SW lineations are parallel to the older, thrust-related lineations. The latter should be turned back towards more southerly-directed directions to integrate corresponding kinematics into the Tethys collisional framework.
Supportive evidence for the Mesozoic age of the tectonic and metamorphic events in the Rhodope Metamorphic Complex has been granted in the recent years, as reviewed in the following paragraphs. Therefore, they must pertain to this geophysically documented, long-lived subduction system.