Interpretation of the Rhodope Metamorphic Complex
The combination of four characteristics of the Rhodope massif lead to a geodynamical model that accounts for simultaneous thrusting and exhumation over a long period of time:
1) A long-lasting subduction is indicated by the large volume of Jurassic, arc-type magmatic protoliths within the Rhodope Metamorphic Complex, and by the Late Cretaceous opening of the Sredna Gora back-arc basin on its northern side [e.g. Boccaletti et al., 1974a; von Quadt et al., 2005]. This subduction was dipping below Eurasia as indicated by the position of the Europe-type remnants of the Upper continental Terrane upon the metamorphic complex and by the S-SSW-directed senses of shear found in pre-Maastrichtian thrust zones. This geological conclusion is reinforced by the tomographic image of a single, northward-dipping slab [Bijwaard et al., 1998], which excludes the Rhodope from being a segment of the European-subducting side of the Balkan-Carpathian system.
2) The metamorphic complex contains a large amount of low-density continental material (marbles, orthogneiss and paragneiss) and a comparatively very small volume of basic and ultrabasic rocks. As argued by Ricou et al. [1998], the buoyancy of the arc and continental material favours decoupling and upward extrusion of such subducted rocks, which then migrate upward with respect to both the hanging wall and footwall tectonic units [Chemenda et al., 1996]. At that stage, thrusting of high-grade metamorphic units over lower grade rocks can be responsible for inverse metamorphic zonation and inversion of synmetamorphic senses-of-shear from floor to roof contacts.
3) The metamorphic Rhodope was eroded to near sea-level by ca. 60 Ma and remained comparatively quiet until ca. 40 Ma. Then, ubiquitous extension began and reached a paroxysm between 35 and 30 Ma.
Island-arc or active continental margin?
The Late-Jurassic granitoids dated in the intermediate units of the Rhodope are products of a magmatic arc. Two questions then arise: (1) was subduction taking place beneath an island arc or a continental margin? (2) Was subduction south- or north-dipping below the Rhodope magmatic arc of that time?
Answering the first question makes use of modelling results on arc-continent collision [Chemenda et al., 2001b; Boutelier et al., 2003]. The accepted interpretation implies an active margin on the northern, European side [e.g. Ricou et al., 1998], and this was based on the intimate association of presumed arc-derived and continental rocks. Now, many more arc rocks have been identified so that the island-arc possibility cannot be discarded without further discussion. Since the majority of the arc-rocks are plutonic, collision systems that would lead to complete arc subduction can be excluded. The lack or scarcity of volcanic and sedimentary sequences also excludes systems leading to accretion of the entire island arc such as the Kohistan [e.g. Bard, 1983; Treloar et al., 1996]. Partial accretion of the island arc addresses the tectonic significance given to the upper crust of the island-arc recognized in the Circum-Rhodope Allochthons of Eastern Rhodope [Bonev and Stampfli, 2008]. One may also wonder whether the gneiss-marble sequences on the northern slope of the arc (Asenitsa-Borovitsa, Figs. 2 and 5) may represent island-arc volcaniclastic and carbonate rocks similar to those seen in today's Bismarck Sea [e.g. Hoffmann et al., 2009]. Separation of the upper crust from the deeper, plutonic crust of the arc now found in the imbricate units involves decoupling within the arc, at the time it became involved in the trench. The possibility of an island arc in the Tethys is tectonically plausible. Collision would have scraped off the upper crust, which remained in low-grade metamorphic conditions. The middle and lower arc crust would have been, at the same time, deeply subducted to sublithospheric "ultrahigh" pressure conditions where they might undergo relatively low temperatures below the thermal shield provided by the subducted arc plate [Chemenda et al., 2001b]. Involving an intra-oceanic arc allows resorption of large amounts of fore-, arc, and back-arc lithospheres, hence more convergence than along a continental margin.
This lack of confident determination between island-arc and continental margin at this stage of the discussion leads us to the question of subduction polarity.
Subduction polarity
The northward sense of shear found in Circum-Rhodope Allochthons of Eastern Rhodope is inconclusive with regard to early orogenic kinematics over a S-dipping slab of the Meliata-Maliac oceanic lithosphere beneath the Vardar oceanic lithosphere [Bonev et al., 2010a]. Since the basal contact is a "scar" with a large metamorphic contrast between high-grade footwall and low-grade hanging wall, the structural argument cannot exclude that the possible island arc has been obducted southward before extrusion of the footwall (hence northward, "scar" shearing in the contact zone). Moreover, and still referring to models [Chemenda et al., 2001a], obduction of an island arc over a north-dipping subduction remains possible. The150-160 Ma age of northward-shearing would date arrival of the continental Lower Terrane in the trench where some back-thrusting could be active. Subduction of that continent would trigger the collisional forces needed to subduct parts of the arc and forearc, and close the backarc [Boutelier et al., 2003; Boutelier and Chemenda, 2008]. This scenario would also be consistent with north-dipping subduction generally inferred in the literature. Consistently north-dipping subduction is also in better agreement with deep tomography, which does not show remnants of a south-dipping slab in the asthenosphere of the region [Bijwaard et al., 1998; Piromallo and Morelli, 2003]. In that case, the Rhodope arc became an active continental margin at the southwestern border of Moesia, the neighbouring part of the European continent [e.g. Ricou et al., 1998; Van Hinsbergen et al., 2005], and this discussion comes back to the point where, concerning the Alpine history, the Rhodope was the active continental margin of Europe, at least during Mid-Jurassic to Late Cretaceous times. Northward subduction is further consistent with marginal basins that were inverted before Albian times in the European upper plate [see discussion and review in Ricou et al., 1998]. The later, Turonian-Santonian rifting in the Sredna Gora back-arc basin indicates the re-establishment of extensional forces in the upper plate, which may indicate slab roll back at that time. In such a reconstruction, the lower terrane was either one of the continental fragments derived from Triassic rifting and migrating away from Africa towards Europe (the Upper terrane) or a continental fragment separated from the southern Eurasian margin during the Permo-Triassic breakup of Gondwana. Like for the Briançonnais in the Alps, closure of Tethys would have rewelded continental fragments of the Early Mesozoic passive margin of Eurasia [e.g. Pleuger et al., 2007].
We are left with some uncertainty concerning the pre-Mid-Jurassic history. For this review, and as far as the Rhodope is concerned, the simplest solution is to consider northward subduction below an active continental margin. The author uses as argument the scarcity of N-MORB ophiolites and the predominance of lherzolite as protolith of most metaperidotites to prefer a continental setting rather than an intra-oceanic setting of the Jurassic arc (Fig. 11). Arc-related magmas of Jurassic age in the Rhodope are evidence for pre-Cretaceous subduction in this part of the Tethys collisional system. This evidence offers a possible correlation with the western contact of the Serbo-Macedonian of the Former Yugoslav Republic of Macedonia, to the northwest [Šarić et al., 2009] and with the Jurassic Pontides, Crimea and Lesser Caucasus arcs known further east [Kazmin et al., 1986; Sengör et al., 1993].
Subsequent subduction of the active margin requires an incoming continent that will be pulled down into the trench. The increasing buoyancy forces of the subducted continental margin can trigger the required torque to produce failure of the overriding active margin followed by subduction of the arc plate [Chemenda et al., 2001b].
Age clusters
Four age clusters (ca 150, 75, 35, <20 Ma) with a possible additional one at about 120 Ma (Fig. 12) are an outcome of the geochronological effort put into the Rhodope over the last decade [see also Liati et al., 2011]. These are, in essence, thermal events recorded by various isotopic systems. The question is whether each corresponds to distinct subduction-collision events thus denoting several subductions of separate terranes [e.g. Liati, 2005]. Such an interpretation neglects the risk that several, often not well understood processes disturb the isotopic systems [Villa, 1998]. This is even true for U-Pb in eclogite zircons [Rubatto and Hermann, 2003]. In this review, the author prefers to understand age clusters in geological units that are linked, as map continuity demonstrates. The author admits hesitation in correlating age cluster and terrane when it comes, in particular, to the Tertiary events of the Rhodope Metamorphic Complex. Several ages taken in the literature as evidence for high-grade, even high-pressure metamorphism are younger than intrusive granites that did not record such metamorphic conditions. Tertiary high-pressure rocks imply extremely fast exhumation rates to be brought to the surface at the time the unconformable sediments were deposited. If exhumation rates are several centimetres per year, one must find the corresponding voluminous amount of erosional products in the proximal sedimentary basins coeval with exhumation. The balance cannot be made in the Rhodope-Aegean region.
The oldest- age cluster is obtained from the ultra-high to high-pressure - high temperature conditions of rocks with older protolith age and of both continental and arc-origin in the imbricate units. Ages span from > 170 to ca 120 Ma (Table 2); 50 Ma is too long a time for a tectonometamorphic event but we note an overlap with magma emplacement (protolith ages) between 160 and 130 Ma in the same imbricate units (Table 1). These two remarks open the possibility that some and oldest ultra-high and high pressure rocks, including melt-bearing sediments and hydrated peridotites, have been carried into the Rhodope Massif by arc magma ascending from an active slab and the mantle wedge. Such a long-lasting, trans-lithospheric process is simulated numerically [Gerya and Meilick, 2010]. Subsequent arc collision and subduction below Europe (Fig. 11) triggered a high-stress collisional regime, which might be responsible for the Late Jurassic-Early Cretaceous (155-130 Ma), intra-continental shortening in the Strandja orogenic belt [Sunal et al., 2011]. The 130-115 Ma sub-cluster may include some high-pressure metamorphic conditions and is therefore considered as the time during which the arc system reached its deepest subduction (Fig. 13). That was immediately followed by the fast upward return of the Rhodope arc to regional, amphibolite-facies metamorphic climax coeval with early, backward crustal stretching at about 110Ma (Fig. 13). The collisional orogen entered then in its waning stage; the 85-60 Ma cluster (Fig. 12, Tables 3 and 7) suits decompression melting, minor plutonism and cooling below metamorphic temperatures of the amphibolite-facies within the orogen, coeval with back-arc magmatism on the European side [e.g. Von Quadt et al., 2003; Georgiev et al., 2009]. The mountain belt was eroded by Late Cretaceous times and earliest sediments were deposited at about 60 Ma (Fig. 14). With this history, we have a simple subduction/collision system, placing extrusion - exhumation of the deeply subducted arc in the Late-Cretaceous and a 110-60 Ma longest-possible erosion period, an acceptable duration in the light of existing mountain belts such as the Alps [e.g. Schlunegger, 1999] and Himalayas [e.g. Najman and Garzanti, 2000]. The orogenic period apparently vanishes until ca. 40 Ma with subordinate magmatism and fault activity in the Rhodopean crust. Although the tectonic picture should be three-dimensional, one may conjecture that subduction and closure of the Vardar Ocean, to the “southwest” of the Rhodope Massif in two-dimensional reconstruction, absorbed plate convergence while mantle delamination and subduction continued. Complexity is met with the younger age clusters. Either geochronological interpretations are correct, and there was a second subduction during the Eocene, or isotopic systems were more or less reset by the large thermal overprint expressed in widespread magmatism. In the first case, the distribution of Eocene-Oligocene ages should display a regional subduction polarity that is not found. Additionally, Late-Cretaceous-Paleocene sediments were not buried and there is no trace of any suture between the basins they represent. The Rhodope massif was deeply eroded and covered by a Late-Eocene shallow-marine cover, which is further evidence for return to isostatic equilibrium of the orogenic area at that time. A potential Eocene subduction and/or resumed subduction zone is at odd with this information.
Figure 12. Histogram of "metamorphic" ages.
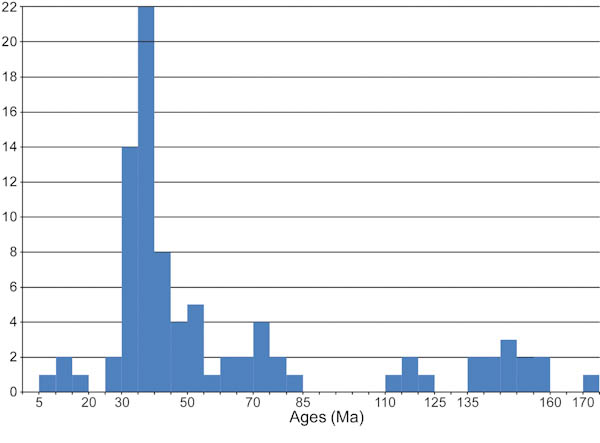
Histogram of "metamorphic" ages reported in the literature for the Rhodope Metamorphic Complex (Tables 2, 3, 4 and 6). Clusters have more significance than number of ages, which largely depends on methods.
Figure 14. Tectonic interpretation across the Rhodope island arc in Late Cretaceous to Oligocene times.
The Late Eocene marks a turning point in the magmatic and tectonic history of the Rhodope. Low-angle detachments demonstrate localized ductile deformation while basins were forming at the surface. The new magmatic cycle that produced acid to intermediate volcanites between 37 and 25 Ma implies melting of the continental crust material. At the same time uplift is documented by the marine beds followed by continental deposits in the related grabens, which indicate crustal extension [Goranov and Atanasov, 1992]. Yet, this event should not have produced much topography as the basins were virtually not eroded since they were formed, which brings suspicion to orogen-forming, collision event. Uplift and crustal extension is a paradoxical association if an isostatically equilibrated crust is accepted for the Late Eocene. Looking for a lithospheric-scale interpretation, as discussed previously, and combining crustal-melting, supra-subduction magmatism, rock uplift, extension and slab retreat since the Eocene-Oligocene, mantle delamination is a plausible solution that has been put forward in other orogens [e.g. the Carpathians, Chalot-Prat and Girbacea, 2000; the Sevier-Laramide, Wells and Hoisch, 2008; the neighbouring Anatolian segment of the Alps-Himalaya collision system, Göğüş and Pysklywec, 2008]. Whilst mantle lithosphere progressively peels away from the Rhodopean crust as a coherent sheet, the delamination point migrates away from its starting point, across the thinned lithospheric region, hence allowing ductile extension of the crust (Fig. 14). Hot asthenosphere flows into the gap between mantle and crust as it opens. Subsequent heating would cause partial melting and magma generation. Magma intrudes the upper crust as post-orogenic plutons and supplies regional, bimodal volcanism while the hot asthenosphere replacing the heaviest lithosphere triggers isostatic uplift and initiates the current topography. Renewed extension in the Miocene may signal the time when mantle peeling reached the oceanic lithosphere behind the collided continental block and started the active Aegean subduction system. This "minimalistic" interpretation in terms of number of subduction-exhumation cycles brings us to the question concerning the present-day crustal structure of the Rhodope.
Crustal structure of the Rhodope
An unresolved structural problem addresses the reconciliation between the large crustal thickness of the central Rhodope, which is over 50 km [Velchev et al., 1971; Dačev and Petkov, 1978; Geiss, 1987] and the large amount of crustal extension expressed in the multiplicity of detachment faults reported in the literature. The 30-50 km deep crust cannot be treated as an old root if we accept that the shallow-marine Eocene cover is evidence for isostatic equilibrium at that time and that mantle delamination generated widespread magmatism in Oligocene times. Abundant magmatic underplating and volcanic “overplating” during the Oligocene is a possible [Isacks, 1988; Kono et al., 1989] yet conjectural process of crustal thickening. Mantle delamination as sketched in figure 14 would result in the thermally weakened and eroded lithosphere as imaged on tomographic profiles [Van Hinsbergen et al., 2005]. Yet, since regional extension reigned in the Rhodope-Aegean region since then, it is unlikely that the present-day 50km thick crust results from compressional shortening of the weak lithosphere, as suggested in the Andes [Beck et al., 1996]. In the quest for an explanation, the dimensions of the Rhodope are too small to invoke lower crustal flow to add material to the crust [Bird, 1991]. One faces the same uncertainty in the interpretation of the thick crust below the Andes where shortening is estimated to be relatively small [e.g. Kley and Monaldi, 1998]. Therefore, the formation of the Rhodope Mountains, with their tectonic specificity, remains an important question to students of orogenic processes.