The results: composition of anatectic melts
The chemical aspects of our research on MI are beyond the scope of this paper. Details concerning the chemistry of anatectic melts in the enclaves from El Hoyazo can be found in Acosta-Vigil et al. (2007, 2010) and in the literature quoted therein, whereas the chemical analysis of MI in migmatites and granulites is still work in progress. Here we provide only a few insights for the potential of this research.
Figure 15. CIPW quartz-albite-orthoclase normative compositions (in wt%) of glasses from El Hoyazo enclaves and KKB and Ronda migmatites
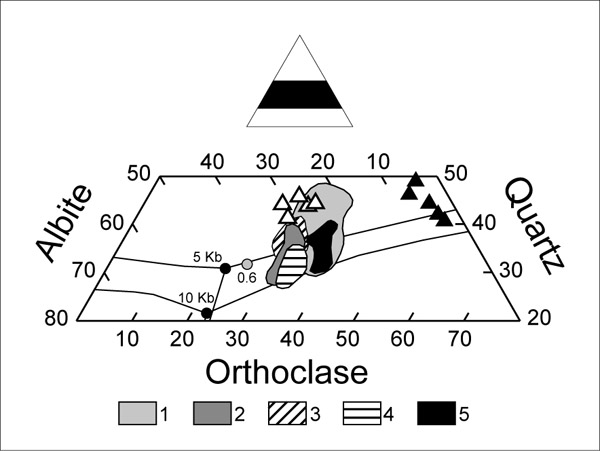
Glasses from El Hoyazo: 1: MI in plagioclase; 2: MI in garnet; 3: MI in Crd; 4: MI in Ilm; 5: matrix glasses. Melt inclusions from the KKB migmatites: filled triangles. Melt inclusions from the Ronda migmatites: white triangles. Black dots and lines in the quartz-albite-orthoclase diagram refer to eutectic points and cotectic lines, respectively, of the H2O-saturated haplogranite system at 5 and 10 kbar. Gray dot refers to the eutectics point of the H2O-undersaturated haplogranite system at aH2O=0.6.
The major element chemical compositions and some trace element concentrations of the MI analyzed so far are summarized in Table 1 (see Appendix A) and Figure 15. Inclusions from both enclaves and migmatites have a peraluminous, granitic composition, in agreement with results from the experimental anatexis of Al-rich metasedimentary parent rocks (Le Breton and Thompson, 1988; Vielzeuf and Holloway, 1988; Patiño Douce and Johnston, 1991). It is important to note that in each of the investigated cases of migmatites/granulites, the KKB and Ronda, the compositions of remelted nanogranites and coexisting glassy inclusions overlap within analytical uncertainty.
Considering the analyses of all inclusions, they show systematic compositional patterns and variations. At El Hoyazo the MI in the different host phases of the enclaves (cordierite, garnet, ilmenite and plagioclase) have slightly different compositions. The integration of textural and chemical information suggests that MI record the progressive entrapment of compositionally evolving melts formed during the prograde anatexis of these rocks. For instance, the composition of MI in plagioclase and garnet indicates relatively low (700-750 °C) temperatures of entrapment and therefore may supply information on the early anatectic history of the rock (Acosta-Vigil et al., 2010).
Conversely, in the KKB granulites, the ultrapotassic and anhydrous compositions of MI in garnets are very far from a “minimum melt” and point to melting temperatures well above minimum or eutectic temperatures, in agreement with the ultra-high temperature (>900 °C) origin of the rock (Cesare et al., 2009). Similar ultrapotassic rhyolites have been obtained by experimental melting of a pelitic protolith at P-T conditions of 5 kbar, 900 °C (e.g., Droop et al., 2003).
Are the chemical compositions discussed above unaffected by post-entrapment processes? In other words, are they representative of primary anatectic melts? Post-entrapment phenomena are well known in MI studies in igneous rocks, and may range from subtle to major: on one side there might be diffusion among the host and the melt or its crystallization/solidification products; on the other decrepitation and opening of the system, with access of fluids through microcracks, may occur; and in-between there is host-melt interaction with dissolution or crystallization (stable or metastable) at inclusion walls. Concerning this latter phenomenon – growth of host within the inclusion – it is important to remind again the major difference in mode of entrapment existing between the MI in migmatites and granulites described here and those from igneous rocks, especially extrusive. As noted above most MI in migmatites are expected to form during melt production, entrapped by a peritectic mineral growing with the melt, whereas MI in igneous rocks form during cooling and are trapped by a host mineral that is crystallizing from the melt. Accordingly, the relationships between solid host and entrapped melt are radically different: inspection of stability phase relationships indicates that when the host is a peritectic mineral, it should not precipitate from the melt within the inclusion. The type and extent of post entrapment modifications can be assessed by careful microstructural observation combined with chemical mapping and analysis. In the glassy inclusions at El Hoyazo, modifications were virtually negligible and occurred by minor crystallization of daughter alkali feldspar at the walls of inclusions hosted in plagioclase (Acosta-Vigil et al., 2007). In the MI in migmatites we expect Fe-Mg diffusion between the garnet host and biotite from the nanogranites. Moreover, more extensive reequilibration might occur during slow cooling if the melt was hydrous. Nonetheless, experimental remelting by the piston cylinder technique, by reproducing P-T conditions close to those of MI entrapment, should drive the melt back (close) to its primary composition.
An additional and important question is whether the composition of MI are representative of that of the bulk melt in the system at the time of MI entrapment. For the case of El Hoyazo, and based on a large number of major (˜300) and trace element (˜50) analyses, Acosta-Vigil et al. (2007, 2010) have shown that MI compositions do not represent exotic boundary layers or local compositions but may correspond to those of the bulk melt regarding major elements and incompatible trace elements. They also recognized, however, that the concentrations of the compatible trace elements were likely affected by local disequilibrium phenomena at the mineral-melt interface, and, therefore were not representative of the bulk melt. More information on this problem requires the acquisition of, and comparison among, large and high quality analytical datasets on many occurrences worldwide.