Petrogenesis and Discussion
Although experimental studies suggest that leucogranites can be generated by a variety of processes, both the major and trace element characteristics of the Bomdila leucogranites are better explained by varying degrees of partial melting and fractionation. An overlap in the wide concentration ranges of oxides, such as SiO2, CaO, MgO, Fe2O3t and Sr (Table 1) of the two-mica and tourmaline granite suites, with no discernable differentiation trends on Harker variation diagram, precludes the derivation of one suite from the other by differentiation following emplacement. This, in turn, suggests that both suites may have been derived from different sources. In order to determine the phase equilibria conditions of the Bomdila granites, the samples were projected onto the Q-Ab-Or phase diagram (Fig. 6) which also contains 2 and 5 kbar minima and eutectics of the haplogranite system with varying aH2O in the melt (Holtz et al., 1991). It can be noticed in the diagram that the two-mica granites do not cluster about a minimum-melt composition characteristic of water-saturated haplogranite phase relations (Tuttle & Bowen, 1958). Instead, they form a spread of compositions with a trend defined by variable quartz/orthoclase and orthoclase/albite ratios. Experimental work by Johannes & Holtz (1990) has demonstrated that quartz/orthoclase ratios increase as pressure decreases in water-undersaturated melts. Further studies on granite indicated that orthoclase/albite ratios of granite minimum melt compositions are greatly increased at decreased water activities (Ebadi & Johannes, 1991). Experimental studies on crystallization of leucogranite magmas (Scaillet et al., 1995) suggest that two-mica granites and tourmaline granites can be generated at low (between 5 and 7.5 wt%) and high (>7 wt.%) initial water contents, respectively. Therefore, the Bomdila two-mica granites with quartz-rich compositions and plotting at decreased pressures (<5 kbars) and low water activities in the diagram, suggest their derivation from an alumina-saturated source under water-undersaturated condition at temperatures >800°C. Whereas the tourmaline granite samples cluster around a composition corresponding to minimum melt at ~3 kbar, aH2O ≈0.5. These values are consistent with thermobarometric studies of the many well-studied leucogranites from the Himalaya such as Manaslu (Guillot et al., 1991), Langtang (Inger and Harris, 1993), etc.
Figure 6. Phase relations and minimum-melt compositions
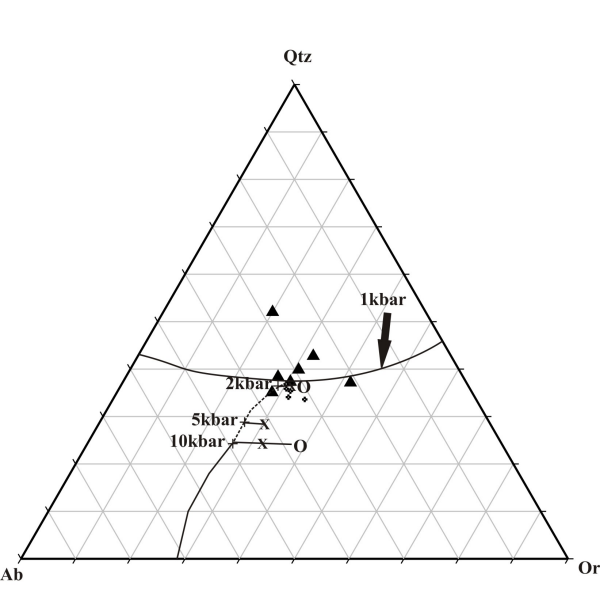
Phase relations and minimum-melt compositions in the system Quartz-Albite-Orthoclase ±H2O. Minimum-melt compositions are from Winkler (1979) and Ebadi and Johannes (1991)., aH2O=1; O, aH2O=0.5; Δ, aH2O=0.3. Qtz= Quartz, Ab= Albite, Or= Orthoclase. Symbols as in figure 2.
Therefore the phase-equilibria considerations and geochemical variation of the two suites of Bomdila gneisses suggest that the dominant petrogenetic process was partial melting rather than large scale post-emplacement differentiation. Further, the peraluminous geochemistry, S-type character, negative Ti, Sr and Nb anomalies of the rocks in primitive mantle normalized diagram and the presence of metasedimentary enclaves strongly support a crustal source, possibly a pelitic protolith for these granites. Harris & Inger (1992) tried to evaluate the melt reactions involved in the generation of granitic melts from pelitic rocks and have given different models such as: 1) fluid-saturated muscovite melting, 2) fluid-absent muscovite melting, and 3) fluid-absent biotite melting. For these models, trace elements such as Rb, Sr and Ba and their corresponding ratios may act as diagnostic. A linear negative trend can be observed in the diagram (Fig. 7), particularly in the Rb/Sr vs. Sr plot and show systematic variation between tourmaline-free and tourmaline-bearing samples. The negative trend clearly corresponds to removal of K-feldspar. The higher concentrations of Ba (up to 952 ppm) in two-mica granites are consistent with biotite melting in the source. The Kd (biotite/melt) value (~6, Hanson, 1978) implies that if more than ~20% biotite is in the residue, most of the Ba from the source will remain in the residue. According to McDermott’s et al. (1996) model, biotite dehydration melting is mainly controlled by the presence of plagioclase or biotite minerals in the source. If the abundance of plagioclase in pelite-dominated sources limits the maximum degree of partial melting, then the melt is enriched in Sr relative to both Rb and Ba. Any increase in the proportion of the greywacke end-member in the source would lower the melt fraction because melting is then limited by the availability of biotite. The melt then becomes enriched in Rb and Ba relative to Sr resulting in high Rb/Sr and Ba/Sr ratios. Thus, it can be inferred from the above discussion that biotite-limited, vapour-absent biotite dehydration melting is the probable mechanism which can best explain the combination of high Rb/Sr, Ba/Sr and Fe2O3 + MgO (up to 11 wt.%) contents of the two-mica granites.
In addition to partial melting, fractional crystallization may also have played an essential role in the generation of two-mica granites as evident from fractionation trends displayed by the granites. For example, decreasing Fe2O3t and MgO (Fig. 2) and increasing Rb/Sr ratios with increase in SiO2 (Fig. 8) exhibited by the granites substantiate the above inference. On the contrary, it is prominently seen that tourmaline granite suite with almost negligible silica range (75–76 wt.%), shows vertical variation in these diagrams, suggesting the insignificant role of fractional crystallization during their formation. The P2O5 abundance will be increased progressively with fractional crystallization in the S-type granites because apatite is soluble in strongly peraluminous and felsic melts (London, 1992). Other elements that occur in phosphate accessory minerals, such as Th, La and Y, decrease in abundance with continuing fractional crystallisation of S-type melts (Chappell 1999), because of the low solubility of monazite. The progressive increasing trend of P2O5 (Fig. 2) and decreasing trend of Th, La and Y shown by the two-mica granites (Fig. 8) further confirms the role of fractional crystallization.
Figure 8. SiO2 vs. Rb/Sr, La, Y and Th plot
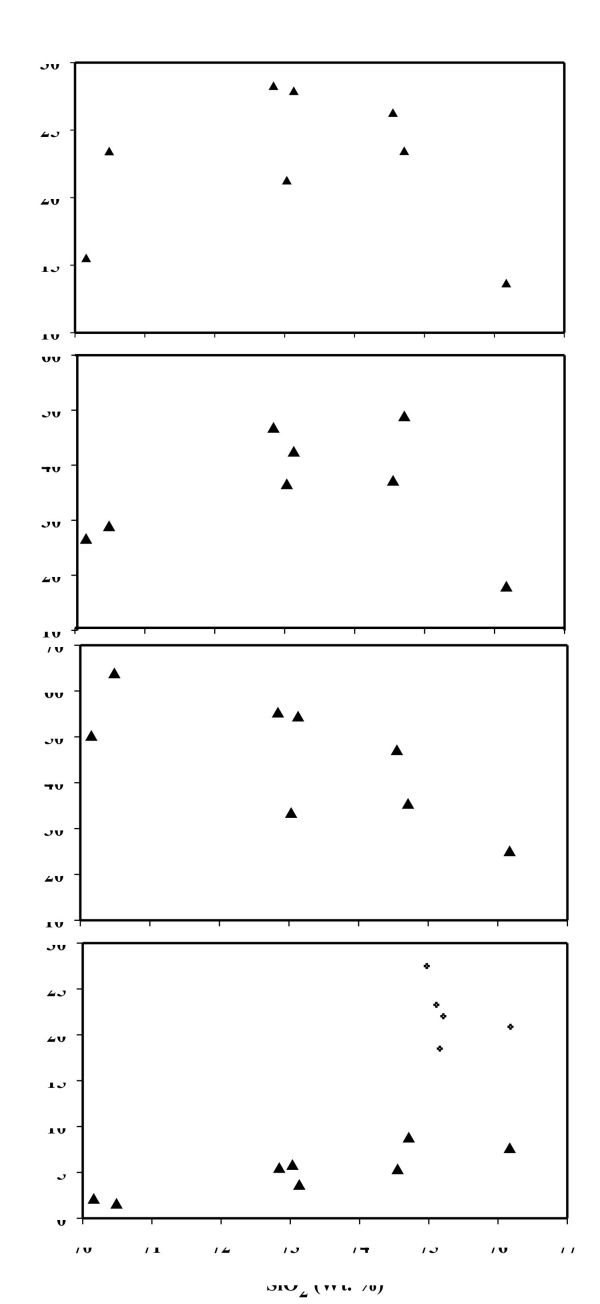
SiO2 vs. Rb/Sr, La, Y and Th plot for Bomdila orthogneisses. Symbols as in figure 2. Note the prominent Rb/Sr positive correlation and negative correlation of La, Y and Th with SiO2 of two-mica granites.
The phase equilibria and geochemistry of the tourmaline granites represent melting conditions of ≥700°C low water activity (Fig. 6) and possibly pressures <5 kbar. The low concentrations of TiO2 (~0.1 wt.%) and Ba in the tourmaline granites suggest a lack of extensive melting of biotite. On the other hand, the high K2O (>5 wt.%), Rb and low CaO (~0.4 wt.%), total iron (~1.5 wt.%), MgO (≤0.5 wt.%) and Sr contents indicate that melts were derived from the incongruent dehydration melting of muscovite (Harris et al., 1993). Various mineral analyses have shown that after tourmaline, muscovite is the most important site for boron in granitic rocks (Rockhold et al., 1987). Dehydration melting of muscovite in pelites is likely to lead to B-rich melts and it has been estimated that 10% fusion of a pelitic schist with 100ppm B will lead to ~1000ppm boron in the melt, enough to produce approximately 3.5% tourmaline (Nablek et al. 1992). This in turn indicates that the petrogenetic process proposed for Bomdila tourmaline granites is consistent with many such other leucogranites from the Himalaya. The ascent of the hot melts of two-mica granites may have triggered low extent muscovite dehydration melting of pelites (most probably schists) higher in the crust producing the boron-rich low-Ti melts (Nabelek et al., 1992) similar to Bomdila tourmaline leucogranites.
From the above discussion, it an be convincingly argued that the two suites of the peraluminous Bomdila orthogneiss were predominantly formed by partial melting and fractional crystallization (in the case of two-mica granites) of pelitic rocks from the upper crust. Presence of metasedimentary enclaves (pelitic schists) in the two-mica granites and the crystallization of magmatic tourmaline in tourmaline granites are also consistent with a metasedimentary source (Bernard et al., 1985). An attempt is made in this paper to explore the possible source rocks for these granites. In this perspective, the mica schists with whom the granites are associated offer potential source lithologies. A striking similarity between the composition of proposed source lithology and the average Bomdila orthogneiss is clearly evident in the diagram (Fig. 9), supporting the assumption that metasedimentary rock (similar to the micaschists) exposed along with granites are the most likely source for the Bomdila orthogneisses.
Figure 9. Trace element abundances in mica schists
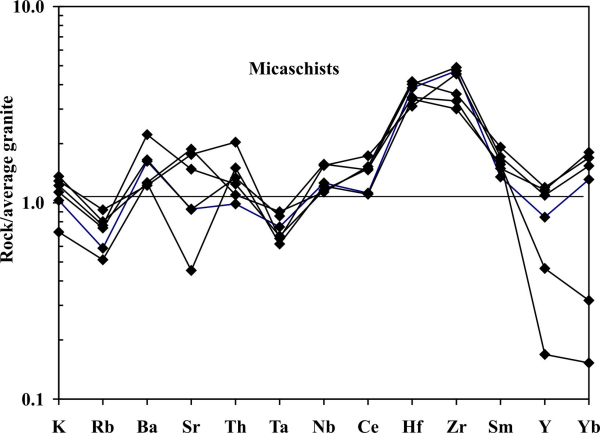
Trace element abundances in mica schists, normalized against average Bomdila leucogranites.