Two centuries of geological history
Two centuries of geological research on the Alps have produced a huge literature body that exponentially grew in the last decades with field and laboratory work, modelling and synthetic interpretations at a regional to global scale. Our histhorical journey begins by briefly recalling older views on Earth’s dynamics, and then moves to the Alps and the evolution from fixist to mobilist concepts. Then it focuses on the period from the late nineteenth to first half of the twentieth century, emphasizing the troubled birth and successful development of the nappe theory, and the genius of those who founded the modern tectonics without knowing the oceanic magnetic reversal, geophysical sounding, petrology, isotopic dating, and other currently availabe facilities. It is also the appreciation of the generally forgotten researchers who provided the first modern geological maps of the Alps.
Older orogenic concepts
In 1785, Hutton presented his “Theory of the Earth” to the Royal Society of Edimbourg (printed in 1788), going beyond the static and biblic views of neptunism. This global theory recognized igneous and other endogenous processes as the essential driving forces of the earth's dynamics, and in this view Hutton is rightly regarded as the founder of modern geology and plutonism (Bailey, 1966). At that time also Lomonosof supported the earth's internal forces and envisaged earthquakes as the principal cause of the sinking and rising of the earth surface (uplift theory; Beloussov, 1980). Hutton and Lomonosof views were ignored by most of their colleagues until, some decades later, Christian von Buch (1774-1853) and Alexander von Humboldt (1769-1859) came to the plutonist conclusion, against Werner (1750-1817) neptunist beliefs, that deep igneous processes did indeed exist and that they were the principal cause of the growth of montain ranges (Dal Piaz, 1997).
In spite of this, the igneous origin of the Mont Blanc granite was recognized only in the second half of the eighteenth century, thanks to Gerlach (1871) and Viollet-le-Duc (1876). It had long been envisaged as a primitive rock and thus termed “protogine” (first-born), the popular name coined by Jurine in 1802. Indeed, the Mont Blanc granite and other gneissic core bodies (crystalline masses according to Murchison, 1849) were wrongly regarded as the oldest rocks of the Alpine basement, a tenet which influenced the debate on the age of metamorphic rocks and the structural setting of the Alps, and was particularly favoured in Italy by Gastaldi (1871) and Baretti (1893).
The growth of mountain ranges continued to be ascribed only to vertical movement (fixist views), disregarding any lateral displacement and crustal shortening. For instance, Von Buch explained the uplift of mountain chains by upward intrusions of igneous bodies and Studer (1851) postulated that rising granite from the interior of the Alpine central massifs could also generate lateral gliding and folding of capping beds. By contrast, Elie de Beaumont (1829, 1852) favoured the view that the orogeny was ruled by cooling and contraction of solid earth.
The contraction theory of Elie de Beaumont was developed by Hall and Dana in the United States and by Suess in Europe. From 1859 to 1873 Hall and Dana introduced the concept of geosyncline, a subsiding basin with sedimentary infilling which would evolve by lateral shortening into the American mountain chains. This model was soon applied by Neumayr (1885), Suess (1893) and Haug (1900) to the former Mesozoic seaway which extended from Gibraltar to Eastern Asia: this basin was named Central Mediterranean by Neumayr and renamed Tethys (the sister and wife of Oceanos) by Suess (1893), pointing out its oceanic features and the concept that the Alpine-Himalayan orogenic sistem was generated from its contractional obliteration (Sengör, 1987, 1990). In 1875, Eduard Suess published Die Entstehung der Alpen, a memoir which greatly influenced the development of modern orogenic views. First, against the plutonist tenet, he sustained the passive role of granitic intrusions during the orogeny, a concept definitively established by Albert Heim (1878). Second, he documented the structural asimmetry of the Alps and their unilateral vergence towards the European foreland, generated by horizontal stresses tangential to the earth's geoid. At the meantime, Heim in his famous work Untersuchungen über Mechanismus der Gebirgsbildung (1878), corroborated the fixist double fold theory early postulated by Escher in the Glarus Alps to explain the presence of older beds over younger ones (Milnes, 1979; Funk et al., 1983).
In 1889 Dutton codified the physical principle of crustal buoyancy (isostasy), later developed by Airy, Pratt and Hayford, and introduced the idea of a plastic-fluid layer below the rigid crust (Hallam, 1995). This model was followed by Suess (1885-1901) in his grandiose work Das Antlitz der Erde, by suggesting that the lighter material, named Sial (Al-rich silicates), rose to the surface during solidification of the earth's molten interior, to form a crust over heavier Mg-rich rocks (Sima) like basalt and peridotite. Crustal contraction during cooling earth continued to be the most likely way in which the mountains and oceans (subsided continents) were formed.
Stratigraphic advances and geological mapping
The principal target of Alpine geologists in the first two thirds of the nineteenth century was the establishment of stratigraphy, the essential starting-point for recognition of doubled or overthrown sedimentary sequences and thereby of thrust nappes. Similar stratigraphic advances also concerned Scotland and Sweden, where the Caledonian nappes could be recognized only once the pre-Ordovician age of the Moines was deciphered (Trümpy, 1996; Butler, 2010).
The first geognostic map of the Austrian empire was producted in 1847, and two years later Franz Joseph founded the imperial-royal Geological Survey, located in the Rasumofsky palace. Between 1864 and 1887, the 21 sheets of the Carte géologique de la Suisse at 1:100,000 scale had been completed (Masson, 1983), including the northern side of the Aosta valley, Piedmont and Lombardy, mapped by Gerlach. The project of a first geological map of the Sardinia Mainland States (Savoy, Piedmont, Liguria) at 1:500,000 scale was entrusted by the King Carlo Alberto to Angelo Sismonda and accomplished in 1862 and 1866 (2nd Ed)(Corsi, 2003, 2007). In the same period Gastaldi and coworkers produced the magnificent Geological Map of the Piedmont Alps (1860-79), consisting of 29 sheets at 1:50,000 scale, hand painted with bright colors (Campanino & Polino, 2002). Never printed, it was spread to the European scientific community at the international Exhibitions of Vienna (1873), Paris (1879) and Turin (1884).
As geological mapping of the Alps developed, the stratigraphy of sedimentary successions was refined and better established. On the other hand, the nature and age of protoliths of metamorphic cover and crystalline basement units long remained uncertain. For instance, Studer & Escher (1839) recognized in Graubünden the anomalous occurrence of granite over a calcschist-greenstone complex (modern ophiolite) but concluded that the former rock (which later studies demonstrated to be older that the ophiolites) was a lateral modification of the greenstone. In the Western Alps, the regular stack of different metamorphic units was regarded by Gastaldi (1871-74) and Giordano (1869) as a normal sequence of three younging-upward layers: the Monte Rosa-Gran Paradiso gneiss and granite was the oldest basement and the overlying “talc-gneiss” (gneissic granite) in the Matterhorn-Dent Blanche region the youngest layer, with the “zone of green rocks” (Piedmont ophiolitic zone) of intermediate age between them. The latter zone was considered by Gastaldi to be Precambrian, whilst its correct Mesozoic age, already envisaged by Elie de Beaumont (1828), Sismonda (1845) and Lory (1873), was definitively established by Franchi (1898) throughout the Western Alps on the basis of paleontological evidence (Sturani, 1975; Dal Piaz & Dal Piaz, 1984).
With the unification of Italy in 1861, the need for a modern chartography showing the lithology, geological setting and resources of the national country led to the foundation of the Royal Geological Service (1862) and the Geological Map of Italy at 1:100,000 scale, a project conceived by Quintino Sella (1862), that started in 1871 and was managed by his friend Felice Giordano (Baldacci, 1911, Caruana et al. 1996; Corsi, 2003, 2007). This project consists of 277 sheets and was accomplished in 1976.
Focusing on the Western Alps, the survey at the 1:25,000 scale of this immense and difficult area (28 sheets at a scale 1:100,000) was performed in a relatively short time (1888-1906) by Franchi, Mattirolo, Novarese, Stella and Zaccagnia, engineers of the R. Mining Corp and then of the R. Geological Survey. A syntheis of this superlative work was anticipated in the Geological Map of the Western Alps at the 1:400,000 scale, published in 1908, four years before the printing (1912) of the first 1:100,000 sheets (Monte Bianco, Aosta, Monte Rosa, Gran Paradiso, Ivrea) of the Aosta valley and surroundings. In the map margin we can read this notice: “According to the engineers Zaccagna and Mattirolo most of the rocks mentioned in this maps as calcschist and specially those with greenstones, together with micaschists and gneiss, would belong to the Precarboniferous instead of the Jura and Trias crystalline facies units”. This advice recalls the fierce scientific dispute between these surveyers, the likely cause of the delay with which the 1:100,000 geological sheets of the Western Alps were printed. Let’s briefly outline the matter of dispute.
Following the intuition of Sismonda and against the opposite view of Gastaldi and Baretti, the Mesozoic age of the calcschists (also labeled schists lustrés) was supported by Lory (since 1857) in the Dora Riparia valley, Favre (1862-67) in the Courmayeur zone, and in part by Gerlach (1869. The Mesozoic age was sustained again by Franchi (1898), documented by the finding of Triassic, Rhaetian and Liassic fossils and extended to the entire ophiolite-bearing Piedmont calcschist-greenstone zone. Franchi’s reconstruction was shared by Haug, Kilian, Revil, Lory, Novarese and Stella and quickly accepted by the plurality of foreign geologists of the time, but were fought by the colleagues Zaccagna and Mattirolo, as mentioned above. The debate was formally settled only in the 1911 when a commission of the Geological Committee, chaired by Torquato Taramelli, approved Franchi’s interpretation for the official geological maps.
Going now to the Italian side of the Eastern Alps in the last decades of the 1800, when Trentino and Alto Adige (Sud Tyrol) were still countries of the Austro-Hungarian empire, the Geologische Bundes-Anstalt of Vienna promoted the geological mapping of the wide area extending from the Ortler massif to the Pusterthal, assigning the survey to a handful of valent geologists. The first survey was provided by Koch, Lepsius, Stache and Teller, but their maps remained unpublished. At the beginning of the twentieth century the Geologische Bundes-Anstalt charged Hammer, Sander and Trener (brother in law of irredentist hero Cesare Battisti) with the mapping ex novo of sheets Bormio und Passo del Tonale, Glurns und Ortler, Nauders and Sterzing of the Austrian Geological Map at 1:75.000 scale, which cover most of western Trentino and Alto Adige. The first three were mapped by Hammer with the contribution of Trener for the Presanella massif, the last one by Sander.
After the war, thanks to a specific agreement between the Magistrato alle Acque of Venice and the Geological Institute of Vienna, these inedited maps were revised and published as sheets Bressanone (1924), Merano (1924) and Passo di Resia (1925) of the Carta Geologica delle Tre Venezie, 1:100,000, edited by the Geological Section of the Idrographic Office of Magistrato alle Acque, directed by Giorgio Dal Piaz (my grandfather). The target of this great project was the survey and printing of 41 sheets: it was accomplished in 1962 and integrated within the Carta Geologica d’Italia at 1:100,000. In this way, Venice gained the regional knowledge of Austrian geologists who, in turn, benefited from Italian funding, when after the tragic war their country was economically exhausted.
Towards the nappe theory
The mobilist revolution developed only in the late 1800s, when some thrust structures were concurrently discovered in the Alps and northern Europe and the nappe theory was conceived (Dal Piaz & Dal Piaz, 1984; Trümpy, 1991, 1996, 2001; Butler, 2010). The starting point was the recognition of inverted sequences and the intuition that, instead of the classic double fold model, this anomaly could even be interpreted through extensive sideways displacements. Unlucky steps in this direction were first attempted by Arnold Escher in the cover sequences of the external Swiss Alps, thanks to fossiliferous sediments and inferred datation, then by Giordano and Gerlach in the extremely more difficult metamorphic domain of the Pennine Alps.
During a field trip in the Glarus Alps, Escher illustrated to Sir Roderick Murchison (1849) the possibility that the occurrence of Verrucano beds over younger deposits was the result of “one enormous overthrow”, a term which recalls the nappe concept. Unfortunately, Escher (1866, in Trümpy, 1991) changed his intuitive idea, favouring the illogical solution of two opposite-vergent anticlinal folds (double fold). Firmly supported by the authority of Albert Heim (1878, 1891), the double fold became a model and was rapidly extended to the Mont Blanc and Gotthard massifs, and then to the Penninic zone and other Alpine domains.
The existence of a large gneissic nappe in the Matterhorn and surrounding mountains - i.e. the Argand’s Dent Blanche fold-nappe - was clearly realized and thoroughly evaluated by Felice Giordano (1869a, 1869b) during his trips around and across the peak (Dal Piaz, 1996a). Giordano's perfect profiles (Fig. 2) show, rightly, that this huge gneissic body overlies everywhere the Mesozoic “green rock zone” (ophiolite) over a distance of at least 35 km, and cannot have risen from below to form a double fold. Therefore, Giordano's crucial question was the age of the capping granitic gneiss (Argand’s Arolla series). If it were older than its sole, the only solution explaining its present location was that “the gneiss of the Matterhorn, Pillonet, and other more distant mountains was overthrown in the form of huge nappes” (Giordano wrote: “..... rovesciatosi poi in falde enormi”, 1869a, and “..... se serait épanché en nappes énormes”, 1869b). Nevertheless, this hypothesis sounded too creative to the rational mind of Giordano, a mining engieneer who favoured the neptunist conclusion (mainly in his French paper, 1869b) that these crystalline rocks were the Mesozoic top of a normal stratigraphic sequence.
Figure 2. Giordano’s and Stella’s geotectonic views.
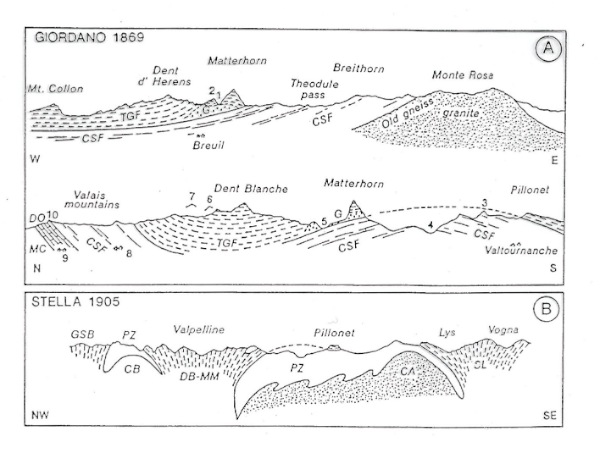
A) Giordano's cross-sections from Valais to the Monte Rosa massif, through the Matterhorn (1869a), showing the continuous overlap of the “talc-gneiss formation” (TGF), including the Matterhorn gabbro (G), over the “calcareous-serpentinite formation” (CSF = green rock zone), in turn resting over the Monte Rosa old gneiss and granite or, to the north, over a dolostone-quartzite suite (DQ) and underlying older micaschists (MC); 1: Lion Pass, 2: Lion Head, 3: Roisetta and Gran Tournalin, 4: Tournanche valley, 5: Stokje, 6: Gabelhorn, 7: Weisshorn, 8: Haudères, 9: Evolene. B) The fixist fan to mushroom fold interpretation of a nearby cross-section along the northern flank of the Aosta valley (Stella, 1905); DB-MM: Dent Blanche-Mt Mary zone, SL: Sesia-Lanzo zone, PZ: Piedmont zone (green rock zone), MR: Monte Rosa basement, AD-BD: Arceza and Boussine domes, GSB: Grand St Bernard zone (Dal Piaz, 1997).
The same hypothesis was envisaged and likewise rejected by Heinrich Gerlach (1869, 1871) who have carefully mapped the whole Pennine Alps and the Matterhorn itself. He believed, rightly, in the older age of the capping protogine-type granitic gneiss, but favoured the fixist double-fold model, disregarding the natural evidence correctly documented by Giordano's profiles. In conclusion, if Giordano's geometry and Gerlach's chronology had been mutually integrated, the Dent Blanche nappe would have been discovered some decades before it actually was by Lugeon & Argand (1905).
Birth and establishment of the nappe theory
After the unlucky attempts at unravelling the structural setting of the Western Alps a stroke of genius was needed to go beyond established concepts. It came from Marcel Bertrand, a brillant French geologist at the Ecole des Mines, who was not influenced by traditional tenets on Alpine orogeny: indeed, he had never worked in the Alps nor visited the Swiss Glarus range but, in 1884, he compared the subsurface structure of the French-Belgian coal-field – his working place - with Heim's classic profile of the Glarus double fold, re-drowning it as one recumbent fold-nappe laterally displaced over a distance of 40 km, the simplest way of explaining the presence of Permian rocks over younger sediments (Trümpy & Lemoine, 1988). Bertrand's paper was the first formal step towards the nappe theory in the Alps, but it was too heretical for overcoming conventional concepts. His mobilist insight was completely ignored by Heim and other influential Alpine geologists, except for Suess (1892), who had never completely convinced by the double fold reconstruction (Trümpy, 1991, 1996).
As pointed out by Trümpy (1991), the factual breakthrough came with Hans Schardt, a professor of geology in Neuchâtel and Zürich. On the basis of a systematic field work, Schardt demonstrated between 1893 and 1898 that some prominent rocks around the Lake of the Four Cantons and the Chablais-Romandes Prealps were exotic remnants of gigantic nappes originating 100 km further to the south-east, later dismembered by erosion to form isolated outliers (Klippen). Gravitational sliding was the favoured mechanism. The importance of Schardt's reconstruction was immediately realized by the geological community, although it was initially criticized. However, the nappe theory rapidly reached almost general acceptance essentially because it was validated by Suess's authority (1901), applied to the whole of the external Swiss Alps by Maurice Lugeon (1902) and finally also blessed by Albert Heim (1902) in a famous open letter to Lugeon. In 1903, a memorable debate broke out at the 9th International Geological Congress in Vienna between the Austrian and German defenders of fixist orthodoxy and the French and Swiss supporters of the new mobilist revolution, a theory which rapidly advanced and became definitively consolidated thanks to the genius of Emile Argand and the authority of Robert Staub. In particular, Pierre Termier (1904), Bertrand's best disciple, successfully extended the mobilist concept to the entire Eastern Alps which suddently lost its traditional autocthony, becaming a stack of basement and cover nappes. Termier (1906) distinguished the first-rank nappes, envisaged as classic recumbent folds, and the second-rank nappes, described as thrust-sheets, developing in the deeper and shallower parts of the orogen respectively, with transitional features between them. This happened some decades before shearing was recognized as generation mechanism of some Alpine basement nappes. Termier conceived the notion of tectonic window, as deep cuts across the eastern Austroalpine tectonic lid of the orogen, where the underlying Penninic units are exposed, and in the meantime Haug (1906) described the cover nappes in the Northern Calcareous Alps.
In the Southalpine hinterland, the fixist fault-controlled staircase structure of the Venetian Alps long sustained by Suess and other reputed geologists was re-interpreted at the century turn by as a typical fold-belt with Adriatic vergence (G. Dal Piaz, 1905, 1912).
Emile Argand and the Penninic fold-nappes
In the early 1900s the Dent Blanche and other nappes of the Penninic zone were revealed and carefully reconstructed throughout the Western Alps by Emile Argand (1908, 1909, 1911). His papers are illustrated with splendid geological pictures, explanatory sketches, tables of cross-sections and tridimensional views which make easier and more immediate understanding Argand’s ideas. His work began with perfect geological mapping at 1:50,000 scale of the Dent Blanche-Matterhorn mountain group (1908, 1909) and was then addressed to the mobilist reinterpretation of the entire arc of the Western Alps, fully represented by a tectonic map, scale 1:500,000, and a series of detailed cross-sections (Argand, 1911a-b). The accuracy of his tectonic map greatly benefited, as was fairly acknowledged by Argand himself, from the perfect fit of the main lithological units, detailed internal subdivisions and mutual boundaries shown in the already mentioned 1:400,000 geological map of the Western Alps, published in 1908 by the R. Servizio Geologico of Italy (Argand said “d’avoir connu, dès sa publication en 1908, l’inestimable document moderne qu’est la carte géologique des Alpes occidentales, au quatre-cent millième, bientôt suivie des premières feuilles alpines, au cent-millième, de la Carte géologique d’Italie, oeuvre distinguée des maîtres du R. Ufficio Geologico”). See Escher & Masson (1984) and Dal Piaz (1996b, 1997, 2001) for reviews of Argand's work on the Matterhorn and the Penninic pile of nappes.
Following Argand's block-diagram (Fig. 3), the axial part of the Western Alps, called the Penninic zone, is a stack of six recumbent fold-nappes, physically continuous and extensively displaced to the north-west, each consisting of old metamorphic and igneous rocks (crystalline basement) regularly mantled by Mesozoic sediments, and locally associated with injections of mafic-ultramafic igneous bodies (ophiolites). Essential features of the Penninic zone are pervasive metamorphism and folding, generated by dynamic crystallization and ductile deformation during the Alpine orogeny. The capping unit is the partly eroded Dent Blanche fold-nappe (VI), which extends from the Valais to the Aosta valley, across the Matterhorn; its origin is recognized in the internal side of the Alpine arc, represented by the Sesia-Lanzo zone (VI'). Below them, are the Mid-Penninic Monte Rosa (V) and Grand St Bernhard (IV) fold-nappes which are separated from the Dent Blanche by a syncline of Triassic marbles, calcschists and greenstones (metamorphic ophiolites) of the Piedmont zone (Giordano's “green rocks zone”). Below them is the Lower Penninic domain, a multiple overthrust system represented by the Monte Leone (III), Lebendun (II) and Antigorio (I) fold-nappes and the Verampio dome (0), the lowermost unit now exposed in the Alps. The Lower Penninic system is restricted to the Ossola-Ticino window, where the nappe stack is domed (tectonic culmination) and the higher nappes have been stripped out; by contrast the higher nappes are preserved in areas of tectonic depression (Valais-Aosta valley to the west and Graubünden to the east). The frontal part of the Penninic nappe stack is thrusted over the Helvetic zone, consisting of decollement cover nappes and the sliced basement of Aar-Gotthard and Mont Blanc massifs; on the opposite side is the Insubric hinterland block (Southern Alps, Po Plain, Dinarides) which, moving north-west, functioned as a rigid indenter against the back of the Penninic nappe pile (the so-called root zone) which was consequently forced to become steeper and bend backwards (Fig. 4). The antithetical slicing of the Southalpine upper crust was an effect of this process.
Figure 3. Argand’s fold-nappes.
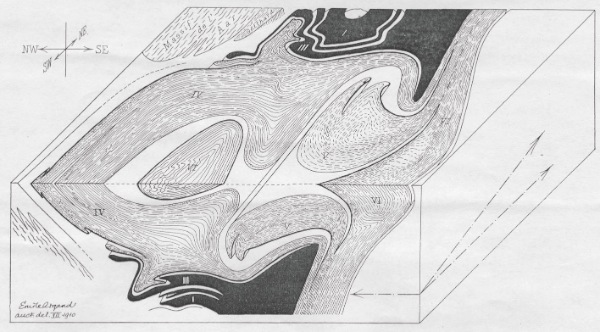
Tridimensional view of the North-Western Alps by Emile Argand. Penninic fold-nappes: Dent Blanche (VI) and its root (Sesia-Lanzo zone: VI'), Monte Rosa (V), Grand St Bernard (IV), Monte Leone (III), Lebendun (II), Antigorio (I), Verampio dome (0).
Figure 4. Argand and the Alpine belt.
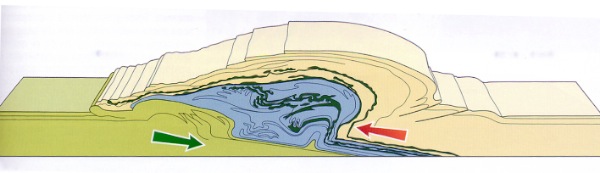
Argand's generalized view of the Europe-vergent Alpine belt. Note that the Eastern Alps (beige) are displaced over the western Alpine nappe stack, and the root zone is indented and back-folded by the Southalpine hinterland, in turn deformed by south-vergent thruSt The Western Alps consist of six Penninic Penninic nappes (blue) and ophiolitic sequences (black), overthrown onto the sliced Helvetic domain and undeformed European foreland (greenish).
In the short work Des Alpes et de l'Afrique Argand (1924a) presented a geotraverse extending from Northern Africa to Central Europe, through the Alps (Fig. 5). This global view shows the collisional structure of the Alps generated by the paroxistic stages of the Tertiary orogeny (Fig. 5a) and a late-orogenic stage dominated by extensional tectonics and crustal stretching to the opening of Ionian basin floored by simatic (mantle) material (Fig. 5b). The Penninic nappe pile and its roots form a flattened zone sandwiched between the rigid toe of the African promontory and the underlying European continent. In addition, if the configuration of the continental crust and its simatic substrate (rich in Mg-silicates) is compared, the essence of two fundamental tenets of Argand's orogenic theory can be appreciated, i.e. contraction-induced crustal thickening and extension-induced crustal downbulge and attenuation at the back of the Alps.
Figure 5. Argand’s Europe-Africa geotraverse and mantle denudation.
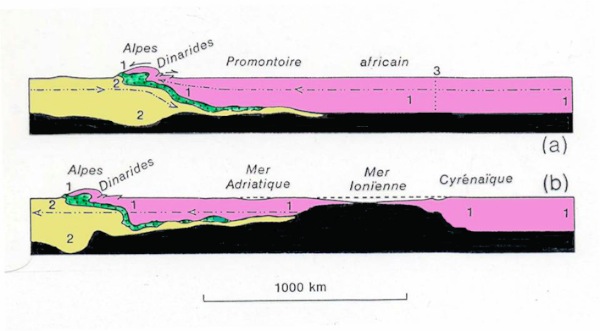
Argand's two-stage reconstruction of a north-south geotraverse from Central Europe to Africa, through the African promontory and the Alps: a) Synthetic structure of the Alpine-Dinarides belt which originated from the collision between Africa (1: pink) and Europe (2: beige), whilst the original Penninic Tethys (green) was shortened and sutured between them; b) Late-orogenic crustal thinning and upheaval of simatic substrate (black), both induced by the northward drift of Europe from a disjunction zone (3), and related opening of Mediterranean and Ionian basins.
Argand (1916, 1924) also reconstructed the kinematic evolution of the Western Alps through thirteen steps of incremental contraction of the Tethyan geosyncline, gradually progressing from embryonic structures, through the generation of second-order geo-anticlines (cordilleras) and synformal depressions (later called Valais and Piedmont basins), towards a pile of recumbent fold-nappes to the ultimate configuration. This evolutionary cartoon was the groundwork for new directions in geodynamics, named embryotectonics, i.e. sequential analysis of the evolving structure (kinematics) and, vice versa, the unrolling of the nappe stack by elimination of overthrusts and shortening (retrodeformation) back to the originally undeformed configuration of Tethys (palinspastic restoration). Large-scale folding was the ductile mode envisaged by Argand to generate the Penninic nappes, supposedly forming a laterally continuous pile of antiformal and synformal recumbent folds. Therefore this view assumes the lengthwise correlation of nappes (cylindristic concept) based on geometry and sedimentary facies development. This view was soon emphasized by Leopold Kober (1923), Rudolf Staub (1924) and Léon W. Collet (1927), who in their great syntheses on the Alps strengthened the genetic link between paleogeographic belts (sedimentary facies zones) and tectonic nappes (Trümpy, 1996).
Argand accepted the essence of Wegener's theory realizing that continental drift could offer driving forces consistent with his kinematic views. Thereby, Argand conceived an innovative model of the Tertiary orogeny in Eurasia and corroborated the concept of continental drift through new geological observations and tectonic reasoning. As a result of his work, Argand was chosen to deliver the inaugural lecture at the 13th International Geological Congress in 1922, where he presented his famous memoir La Tectonique de l'Asie (published in 1924) in which the mobilist concept was thoroughly tested and definitively consolidated. Dealing now only with the evolution of the Alps, it can be stressed that Argand partly abandoned his previous belief (1916) in a continuous orogenic contraction of Tethys from the Carboniferous to the Neogene and suggested the existence of intervening extensional pulses, chiefly in the Triassic-Jurassic geosyncline times. Crustal extension and thinning were envisaged also in a late-orogenic stage, giving rise to the opening of the modern Mediterranean and Ionian basins, locally floored by simatic material. Crustal stretching was supposedly induced by the northward drift of Europe and its moving away from a disjunction zone located to the south. This mode of crustal attenuation provided a suitable analogue for the generation of the Alpine geosyncline, the cradle of the orogeny, where the Penninic zone could play the role of the mobile geosyncline core. In this view, Argand (1924) innovated his previous belief on ophiolites as syn-orogenic injections of mafic melts (1916), partly envisaging the modern notion of crustal tearing and mantle denudation. This promising direction was no longer developed and, in 1934, Argand went back to the injection concept in his conclusive work La zone pennique (Escher & Masson, 1984).
In the meantime Kober and Staub published their synthetic reconstruction of the entire Alpine chain. Kober (1923) fully accepted the nappe theory, but envisaged a symmetrical structure of the Alps thought to be generated by a double thrusting mechanism, with crustal attenuation of the axial zone (Fig. 6) and a reduced extent of the bilateral nappe displacement. Staub (1924) referred the Austroalpine nappe stack to the front of the Adriatic-Apulian promontory, detached from its Southalpine hinterland and thrusted over the ophiolite-bearing Pennine nappes, which in turn override the continental units of the Helvetic external domain. Based on an extremist use of cylindristic canons, Staub proposed the eastward and physical continuous extension of the western Penninic nappes beyond the Ossola-Tessin dome and their reappearance in the Grisons and Hoen Tauern area below the Austroalpine basement and cover nappes (Fig. 7). His memoir is illustrated with a tectonic map of the Alps, one million scale, and three color plates of transversal and longitudinal profiles.
Figure 6. Kober’s bilateral thrusting.
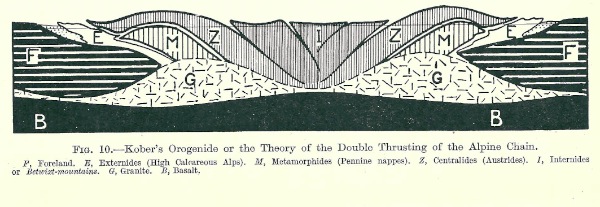
Kober's Orogenide theory of double thrusting of Alpine chain (in Collet, 1927). Structural elements: Foreland (F), Externides (E: High Calcareous Alps), Metamorphides (M: Pennine nappes), Centralides (Z: Austrides), Internides (I), granite (G), basalt, sima (B).
Figure 7. Staub’s longitudinal correlations.
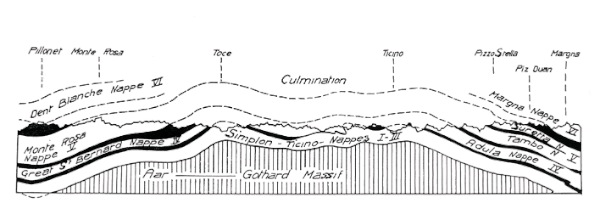
Staub's longitudinal correlation of Alpine nappes on both sides of the Simplon-Ticino culmination (in Collet, 1927).
Criticism and further advances
The tectonic views of Argand and Staub dominated the Alpine scenario until the end of the 1960s, with some fixist reactions (gravitational tectonics) and some innovative contributions on the debated mechanism of nappe generation. Opposite to Argand’s fold-nappes, also emphasized by Albert Heim after his mobilist conversion (1902), Staub (1937) and the lesser known Austrian geologists Ampferer and Hammer (1906, 1911) recognized in the central and eastern Alps the existence of thrust-planes at the base of most nappes, instead of the alleged reversed limbs. As pointed out by Trümpy & Oberhauser (1999) and Trümpy (2001), it can be mentioned that Otto Ampferer (1906, 1911) introduced the notion of underthrusting or subfluence (Verschluckung) of crustal slices into the interior of the Alpine edifice, before the term subduction was coined by Amstutz (1955) in the Ossola valley, without any plate tectonic meaning. Ampferer’s innovative ideas were dispersed in short notes that were little understood or ignored by his contemporaries.
Since the 1930s, a modern description of the tectono-metamorphic evolution of the Italian Eastern Alps were made by Angelo Bianchi (1934) and Giovanbattista Dal Piaz (1934), based on detailed mapping, structural analysis, laboratory work and mobilist views, whilst the personality of Sander and Cornelius emerged among other distinguished Austrian geologists, dealing with the fabric of the rocks, the Eastern Austroalpine and the Tauern window.
Back to the Western Alps, it is worth mentioning that in 1925 Hermann proposed a provocative restoration of Argand's Penninic zone, envisaging the Dent Blanche, Monte Rosa and Grand St Bernard nappes as deriving from individual continental fragments - named microcratons - scattered within the Tethyan geosyncline between the European and African continents, and alternating with deep marine basins floored by simatic crust, the source of the Alpine ophiolites. Note that this forgotten palinspastic reconstruction does not much differ from Trümpy’s (1980) and Platt’s (1986) classic cartoons. Modern descriptions of petrographic and chemical features of Piedmont ophiolites and related eclogites were given by Zambonini (1906) in the Cottian Alps and by Gb. Dal Piaz (1928) in the Aosta valley.
From 1937 onwards, Staub displaced Argand’s Dent Blanche nappe from the Penninic to the Austroalpine domain, hence to Africa, owing to the lithological affinity clearly documented by the surveyors of the Geological Map of Italy. In 1938 the Swiss students Stutz and Masson replaced the Dent Blanche fold-nappe, including its Mesozoic envelope, with gliding thrust sheets bounded by mylonitic shear zones. In spite of this, Argand's concept of fold-nappe generation by primary foldings was long maintained until, from the early 1970s onwards, it came to be severely criticized.
Alpine geology after World War II
After the war, Alpine geology was gradually reappraised, leading to significant improvements in field work, stratigraphy, structural geology of sedimentary cover units, and retrodeformation of the Alpine nappe stack into various paleogeographic scenarios (e.g., Ellenberger, 1953, 1958; Elter, 1960; Trümpy, 1960; Tollmann, 1963, 1977). In particular, Ellenberger (1953) recognized the Briançonnais affinity of the Barrhorn series (Fig. 8), previously included in the Piedmon zone, and of similar sequences in the Mediane Prealps.
Figure 8. Briançonnais series near Zermatt.
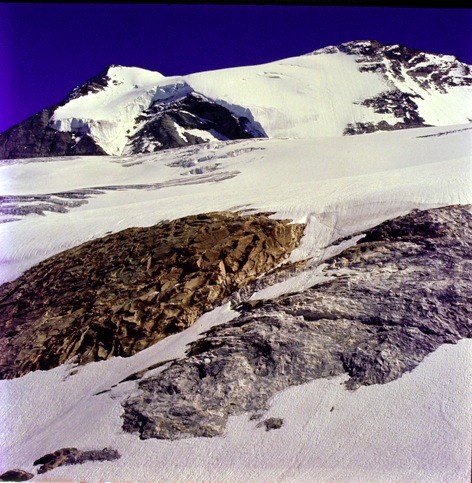
The Barrhorn series, near Zermatt, displays a Briançonnais affinity (Ellenberger, 1953; Sartori, 1987, 1990) and is interpreted as the detached cover of the Siviez-Mischabel (Grand St Bernard) nappe. This figure shows a typical occurrence of white pelagic marbles (Malm) and yellowish impure marbles (Cretaceous), and the overlying Dent Blanche nappe (Arolla gneiss); the ophiolitic Combin zone, running between them, is hidden beneath the Turtmann glacier.
The geosyncline concept continued to be developed by regional reconstructions of Tethys from the Mediterranean to the far east (Stille, Aubouin and co-workers; in Aubouin, 1965). By contrast, the understanding of geodynamic processes went through a period of relative stagnation, characterized even by the exhumation of neo-autochthonist models supported by some French and German geologists. In the late 1950s and 1960s, the principal lines of Argand and Staub's work were generally accepted in the Alps and corroborated by detailed regional studies, so a sort of mobilist Renaissance may be recognized (Trümpy, 1996).
From the early 1970s onwards, the fold-nappe model was severely criticized, since the recumbent folding was shown to be a post-nappe deformation and the existence of decollement and shear mechanisms was revealed for most of the Alpine nappes (Milnes and his students, in Dal Piaz & Gosso, 1984). It can also be recalled that Milnes (1974, 1978) in his pioneering works suggested the Sub-Penninic affiliation of the lowermost Penninic nappes exposed in the Ossola-Tessin window, including also the Gotthard and Tavetsch massifs traditionally referred to the Ultrahelvetic-Helvetic domain (Spicher, 1980; Bigi et al. 1990). Mesozoic normal faults inside Tethys and the contrasting evolution of individual fault-bounded units were discovered across the Alps, whilst controversial amounts of crustal shortening were suggested through retrodeformation of some Alpine nappes. In the Eastern Alps, nappe emplacement and concurrent metamorphic imprint were anticipated to the Cretaceous by Flügel (1960) and other Austrian geologists, owing to the relation between the Austroalpine thrusts and sealing Gosau sediments (Oberhauser, 1980, 1995, and refs. therein). In the early to mid-1960s geochronological data on igneous and metamorphic rocks started to emerge in the Alps (Hunziker et al., 1992, and refs. therein) and thereby new constraints were established on the orogenic evolution at depth. Meantime, field surveys, theoretical groundwork, laboratory experiments and geophysical techniques greatly expanded in Europe and all over the world.
Plate tectonics and its impact on Alpine geology
It is well known that the global theory of plate tectonics was conceived in the 1960s through the contemporary contribution of a handful of brilliant scientists and research teams. Its conceptual birth goes back to the American geologists Harry Hess and Robert Dietz, the English geophysicist Fred Vine and some distinguished precursors, whilst it is generally acknowledged that the theory was promoted and established by geophysicists such as the Canadian Tuzo Wilson, the Americans Jason Morgan, Bryan Isacks, Jack Oliver, Lynn Sykes and the French Xavier Le Pichon (Hallam, 1973; Bosellini, 1978).
This global theory of earth's dynamics developed outside the Alps in spite of the decisive contribution they had provided for centuries to fundamental advances in the orogenic concept. It suddently appeared to the world of Alpine geology in the latest 1960s and its impact was not welcomed enthusiastically. Like Venus, this theory born out of the sea (Trümpy, 2001), far from the classic highland geology of the Alps, and clearly it was to dismantle the latest refinement of the geosyncline model and other traditional milestones, ignoring any Alpine inheritance for the mobilist tenets and collisional features. Actualist reconstructions were mostly provided by outsiders who were not introduced enough into, or not biased by, any complex details of the Alps. By contrast, partial remakes or quite heterodox models were chiefly suggested by Alpine geologists induced to privilege each alleged regional constraint over potential modern homologues. Personal study areas were aften regarded as a special case where only plate tectonic canons fitting regional data could be applied, whereas those supposedly contrasting them were discarded or ignored.
Laubscher (1969, 1970) depicted two opposite subductions converging down into a mutual vertical root (Fig. 9), inspired by the crustal downbulge envisaged long before by Hess (1939). In the meantime global models of Tethyan evolution were suggested by Dewey & Bird (1970), Smith (1971) and Dewey et al. (1973). The sialic geosyncline became an immense basin floored by a basaltic crust and laterally bounded by faulted and attenuated passive continental margins, whilst ophiolites become remnants of lost ocean floors (see Bernoulli & Jenkyns, 2009, for review). A modern reconstruction of various mountain belts in the world was given by Dewey & Bird (1970), particularly evaluating the closure of the Tethyan ocean and continental collision in the Alpine-Himalayan realm, a concept quite obvious in Argand's and Staub's models. The evolution of the Atlantic ocean and relative motion between Africa and Europe were computed by Smith (1971) and Dewey et al. (1973); this allowed the recognition of a left-lateral fault zone in the Mediterranean until 90-80 Ma ago and of NW-trending oblique convergence (of about 800 km) from the Late Cretaceous to the Present. In this view Europe became the lower plate since the onset of subduction. All ophiolitic units of the Alpine, Apenninic and Hellenic belts were immediately reinterpreted as lithospheric remnants squeezed out from the suture of western Tethys oceanic branches separating the European and African continental margins (Laubscher, 1969, 1971; Dercourt, 1970; Boccaletti et al., 1971; Dal Piaz, 1971, 1974; G. Elter, 1971; Hsü & Schlanger, 1971; Wezel & Ryan, 1971; Dal Piaz et al., 1972; Haccard et al., 1972; Lemoine, 1972, 1975, 1977; references and review in Abbate & Bortolotti, 1984). In particular, the Alpine-Apenninic (Piedmont-Ligurian) ocean was envisaged as a rhomboidal basin dominated by strike-slip deformations, where thinning and tearing of the continental crust progressed up to its complete splitting, leading to mantle denudation (Decandia & Elter, 1969; Elter, 1972). In this view, a pre-oceanic process of gabbro underplating was figured by Elter (1972) in a pure-shear thinning model of the Ligurian domain.
Figure 9. Laubscher’s opposite subductions.
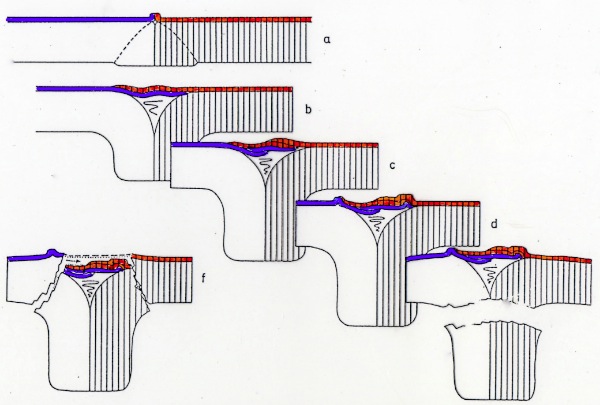
Opposite subductions of Africa (red) and Europe (blue), converging down into a mutual vertical root (Laubscher, 1969, 1970), inspired by Hess (1939) crustal downbulge.
At the beginning of the 1970s, the convergent plate margins were thermally modelled (Oxburg & Turcotte, 1970) and the blueschist to eclogite facies metamorphism, recognized in the Alps since the late 1800s (e.g., Stella, 1984, 1903; Franchi, 1895, 1897, 1902; Novarese, 1985; Frey et al., 1974, and refs. therein), became the signature of fossil subduction zones around the Pacific ocean and in the Alps (Ernst, 1971; Dal Piaz, 1971, 1974; Dal Piaz et al., 1972) (Fig. 10). The Sesia-Lanzo zone, some basement slivers of the Dent Blance nappe s.l. (called outliers), and the underlying Piedmont ophiolitic units were a key for understanding the subduction metamorphism in granitoid, pelitic and mafic rocks, its timing, and the exhumation of high-P units from mantle depths.
Figure 10. Subduction metamorphism in the Western Alps.
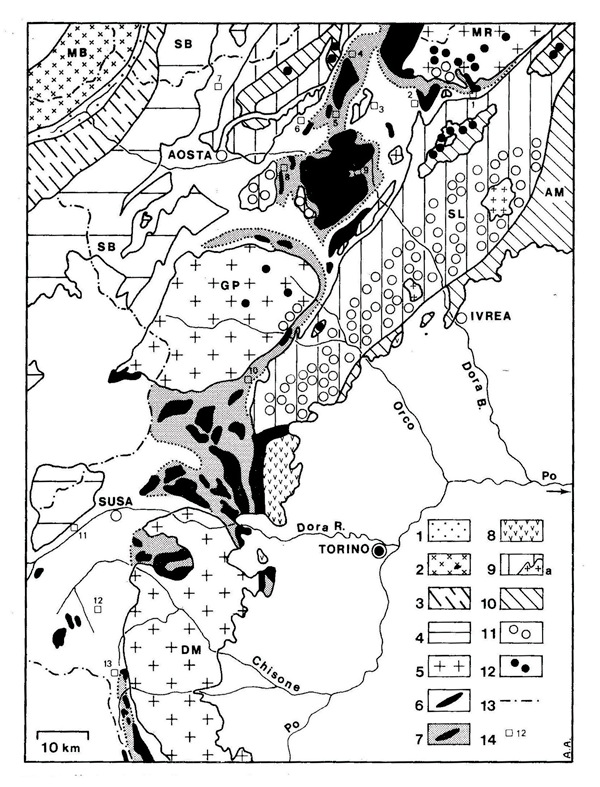
Tectono-metamorphic map and geodynamic evolution of North-Western Alps (Dal Piaz, 1971).
A) Map - Helvetic-Ultrahelvetic zone: sedimentary cover units (1) and crystalline basement (2); Subbriançonnais zone (3); Grand St Bernard nappe (4); Penninic crystalline units (5) of Monte Rosa (MR), Arcesa-Brusson, Gran Paradiso (GP), Dora-Maira (DM); Piedmont zone, including prasinite-bearing calcschist units (6), and ophiolite-rich eclogitic units (7), with major serpentinite bodies (black); Lanzo lherzolite (8); Sesia-Lanzo zone (SL) and Dent Blanche Arolla series (9), intruded by late Alpine Biella-Traversella plutons (9a); 2nd Diorite-kinzigitic zone and Valpelline series (10); probably Alpine eclogitic imprint in the Sesia-Lanzo, Dent Blanche, Monte Rosa and Gran Paradiso (11); eoalpine kyanite-chloritoid ± glaucophane assemblages (12); national boundaries (13); Cu-Fe ore deposits (14).
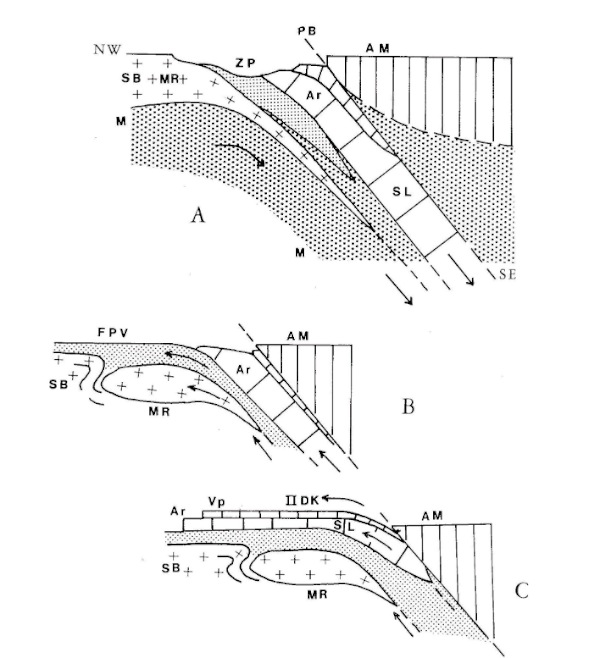
B) Profiles – Eoalpine subduction (A) of a composite plate, involving the Grand St Bernard (SB), Monte Rosa (MR), Piedmtont sediments and ophiolites (ZP), upper mantle (M) and probably the Arolla series (AR), Sesia-Lanzo zone (SL), Valpelline series (VP) and 2nd Diorite-kinzigitic zone (IIDK), below the continental basement of the Souther Alps (AM); Benioff plane (PB); upflit and nappe emplacement (B-C).
It can be remembered that, when the memoir on the Sesia-Lanzo zone was printed (Dal Piaz et al., 1972), this classic eclogitic metamorphism was firmly tought to be pre-Permian (Bianchi & Dal Piaz, 1963; Gb Dal Piaz, 1965), pre-Carboniferous (Carraro, 1966, 1972) or even Caledonian (Mottana, 1972), due to the alleged Permian age of post-metamorphic porphyritic dykes and volcanic agglomerates of the Sesia-Lanzo zone. It can also be remembered that, at the beginning of the 1970s, the tectono-metamorphic evolution of the Western and Central Alps was considered as a single polyphase cycle of burial type and Tertiary age only (e.g. Niggli, 1970; Trümpy, 1971). The breakthrough could have been an oral presentation held by Hunziker (1970) in a Swiss meeting, but new dating was strongly contrasted by an influential Swiss geologist and the paper was not puplished. A new collaboration started and the field and laboratory work was improved. Two years later, the innovative Rb-Sr and K-Ar ages on white micas from eclogitic granitoids and micaschists of the Sesia-Lanzo zone and their geodynamic interpretation were presented to the Società Geologica Italiana and published (Dal Piaz et al., 1972), supporting the existence of a subduction-related tectono-metamorphic event of Late Cretaceous age (90-65 Ma; detailed isotope data in Hunziker 1974). Criticism vanished with finding and dating (31.6 ± 1.3 Ma; Dal Piaz et al., 1973) of a post-metamorphic lamprophyre dyke across the contact between the Sesia-Lanzo and the Combin zone, near the Passo Palasina (Ayas valley), and with the extension of this chronology to the identical dykes (previously Permian) that constrain the upper age limit of the eclogitic metamorphism (32-30 Ma; Dal Piaz et al., 1977). The eclogitic Sesia-Lanzo zone rapidly became the best evidence that also large and coherent slices of light continental crust could be deeply dragged down in the subduction zone (Dal Piaz et al., 1972; Compagnoni et al., 1977), contrary to theoretical views that ruled out the subduction of continental crust due to its buoyancy. The interest for this topic allowed the development of the triennial “Italy-U.S.A. cooperative project on high pressure to low temperature metamorphism in the Western Alps”, funded by C.N.R. and N.S.F., and lead by Robert Coleman, Gary W. Ernst and myself. The first issue was the internal report “The Sesia-Lanzo zone, a slice of continental crust with Alpine high pressure-low temperature assemblages in the western Italian Alps” (Compagnoni et al., 1975) that was distributed to all participants at the Special session of the Soc. Italiana di Mineralogia e Petrografia on “High Pressure-low temperature metamoprhism of the oceanic and continental crust”, held in Genova 23-29 September 1976, and re-printed the year later (Compagnoni et al., 1977).
The kinematic modelling and paleostructural restoration of the Alpine nappe stack, traditionally inferred from facies analyses of cover sequences, became to be extended to deeper crustal levels, and integrated with structural, petrological and isotope data from tectono-metamorphic units. When these powerful tools to trace and date the vertical component of kinematic trajectories were properly evaluated, each evidence from metamorphic continental and ophiolitic nappes contributed to reconstruct the tectono-thermal evolution of the Western Alps (Dal Piaz et al., 1972; Compagnoni & Maffeo, 1973; Bocquet, 1974; Frey et al., 1974; Hunziker, 1974; Pasquarè, 1975), and the Eastern Alps, from eoalpine subduction to mesoalpine collision and thermal restoration (Oxburgh, 1972; Oxburgh & Turcotte, 1974; Hawkesworth et al., 1975; Dietrich, 1976).
Exhumation of eclogitic nappes, a recurrent matter of debate, immediately appeared more problematic than their subduction at depth. Buoyancy-dominated uplift (Ernst, 1971) or forced upward movement of imbricated slices, moving parallel to and over the still active subduction zone were hypothesized (Dal Piaz et al., 1972; Hunziker, 1974). The latter mechanism was favored because surface uplift and erosion appeared inadequate to produce the required unloading, and also because the Oligocene-Miocene molasse was deposited after most of the exhumation had already been accomplished. This is demonstrated, for instance, in the Sesia-Lanzo zone, where Oligocene clastic and volcanic sequences discordantly cover the eclogitic basement units (Dal Piaz et al., 1972; Sturani, 1975).
Mountain building in the Eastern Alps was interpreted with an innovative model based on flake tectonics and an unusual northward subduction of the southern plate (Oxburgh, 1972; Oxburgh & Turcotte, 1974): in this view, collisional crustal thickening was generated by a southward indentation of the European continental prong (Tauern and Bohemian massifs), wedging apart the Austroalpine nappe system and the underlying delaminated mid-lower lithosphere of the southern African plate (Fig. 11). Such possibility was soon ruled out. Crustal delamination was broadly utilized by Hawkesworth et al. (1975) in a kinematic model carefully accounting for the polyphase metamorphic signatures (Oxburgh & Turcotte, 1974; Bickle et al., 1975). Paired metamorphic belts and a thermally-controlled delamination of the Adria upper plate were suggested, and supposedly generated by the colliding continental crust of the European lower plate: the upper crust of Adria overrides the flysch-ophiolite suture, forming the Austroalpine nappe system, whilst its mid-lower lithosphere is pushed away to the south, beneath the Southalpine domain. This reconstruction supported the Cretaceous closure of the Tethyan ocean, suggesting that major changes in the Europe-Africa movement likely occurred in the Lower Cretaceous, contemporaneously with the opening of southern Atlantic (Hawkesworth et al., 1975; Fig. 11).
Figure 11. Plate tectonic models in the Eastern Alps.
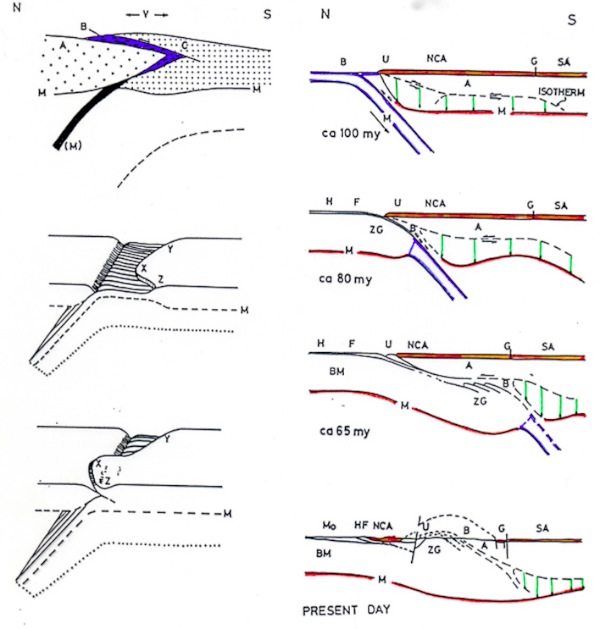
Left - Flake tectonics and northward subduction of the southern plate (Oxburgh, 1972): Bohemian massif and Tauern gneiss (A), ophiolite-bearing calcscshists (B), Austroalpine nappes (C), Moho (M), oceanic crust (black), base of northward subducting African lithosphere (dotted line).
Right - Mesozoic-Tertiary evolution of the Eastern Alps (Hawkesworth et al., 1975), with continental collision and thermally-controlled delamination of the Adria upper plate: Austroalpine old basement (A), ophiolte-bearing calcschists (B), Bohemian massif (BM), flysch (F), Gailtal line (G), northern limit of Sauthern Alps (SA), Lower Austroalpine (U), Northern Calcareous Alps (NCA), Penninic Central gneiss inside the Tauern window (ZG), Helvetic zone (H), Molasse basin (Mo), Moho (M).
Field work and isotope dating (Borsi et al., 1973, 1978; Sassi et al., 1974) provided new constraints to the evolution history of the upper Austroalpine polymetamorphic basement (Mules-Merano complex, Baggio et al., 1969), south of the Tauern window. The basements is subdivided in two blocks by the Deferegger-Anterselva-Valles fault (DAV), early depicted by Gb. Dal Piaz (1934) in his Carta geo-tettonica dell’Alto Adige orientale, 1: 200,000 scale: whilst the southern block displays only Variscan isotope ages, the northern one is characterized by an eoalpine (Cretaceous) overprint, as evidence of differential uplift between them (details in Mancktelow et al., 2001).
At the end of the 1970s, the appeal of the new global tectonics among Alpine geologists is documented by a growing number of palinspastic and kinematic reconstructions of the collisional belt, as a whole, or of its signifcant sections, and by reviews (e.g., Dal Piaz, 1974; Closs, 1975; Debelmas, 1975, 1976; Dietrich, 1976; Dietrich & Franz, 1976; Roeder, 1976; Compagnoni et al., 1977; Frisch, 1977, 1979; Bickle & Hawkesworth, 1978; Caby et al., 1978; Lombardo et al., 1978; Mattauer & Tapponnier, 1978; Milnes, 1978; Roeder & Bögel, 1978; Tollmann, 1978; Gosso et al., 1979; Hsü, 1979).
Figure 12. One Moho below the Western Alps.
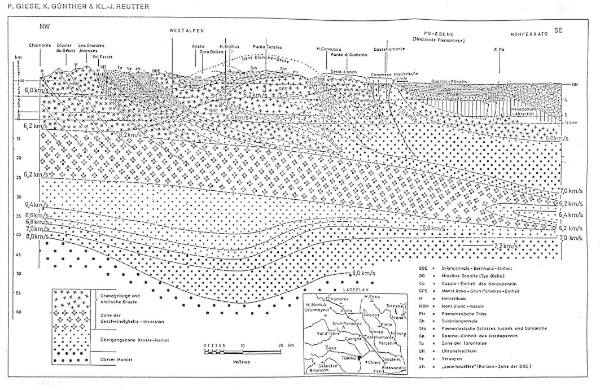
Seismic transect from Chamonix to the Po plain (Giese et al., 1970), nearly parallel to the CROP-ECORS profile, showing a thickened collisional crust (55 km) that includes the high-velocity Ivrea body and is underlain by a physically continuos Moho.
A crustal downbulge in the Alpine belt had long before been recognized by classic gravity modelling and seismic surveys whilst, as previously recalled, the existence of deep roots was hypothesized by Laubscher (1969, 1970). This insight was a direct consequence of kinematic constraints imposed by a two-sided subduction model supposedly involving equal amounts of lower lithosphere of the African and European plates, both converging down towards a vertical root. The existence of Alpine roots appeared to be confirmed when a relatively dense rigid body was detected inside the asthenosphere in the internal part of the Alpine arc, through seismic surface and body waves (Panza et al., 1980) or teleseismic residuals (Babusca et al., 1987). It should be pointed out that, till the late 1980's, the thickened collisional crust was thought to be underlain by a unique and physically continuos Moho, extending from the foreland to the hinterland (Giese et al., 1970, Fig. 12; Angenheister et al., 1975; see Roure et al., 1990, for review).
Towards the end of the Twentieth Century
Significant advances of theoretical and laboratory research extended their benefic effects also to the Alps, whilst new structural and petrological investigations were systematically addressed to the continental and oceanic units of the collisional belt. The geochronological data base was noticeably enlarged (SIMP, 1985; Frank et al., 1987b; Hunziker et al., 1992, and refs. therein). The importance of a multi-disciplinary approach for unravelling the Alpine puzzle and its tectono-thermal evolution was definitively asserted, even if only little effort was devoted to harmonically integrate these constraints with data inferred from cover sequences and geophysical soundings (and vice versa). A new Structural Model of Italy - six maps at a 1:500,000 scale - was elaborated by the Italian Geodynamic Project (CNR); sheets 1-2 (Bigi et al., 1990) and 3 (Bigi et al., 1993) cover the entire Alpine chain, and are discussed in the second part of the paper.
For clarity, principal advances in the last decade of the Twentieth Century are grouped in some general topics, as follows.
Tectono-metamorphic evolution
New or better calibrated petrologic estimates progressively allowed new attempts at reconstructing the kinematic trajectories followed by individual tectonic units from the ocean floor or passive continental margin to the present surface, through the subduction thermal low, subsequent collisional relaxation and related polyphase deformation. This evolution is recorded by a few P-prograde relics, generally preserved inside eclogitic garnets, the eclogitic peak and a sequence of P-retrograde mineral transformations recognized in low-strain domains from oceanic and continental nappes of the Alps (Spalla et al., 1996, and refs therein). Finite strain under different P-T regimes was investigated in the eclogitic Sesia-Lanzo continental crust (Pognante et al., 1980; Passchier et al., 1981; Lardeaux et al., 1982; Williams & Compagnoni, 1983) and other domains (e.g., Ramsay & Allison, 1979; Milnes et al., 1981; Ayrton et al., 1982; Choukroune & Gapais, 1983; Steck, 1984; Ring et al., 1988). The displacement of individual nappes or metamorphic coherent groups of nappes became to be inferred from shear indicators and stretching lineation patterns (e.g., Malavieille et al., 1984; Baird & Dewey, 1986; Choukroune et al., 1986; Lacassin, 1989; Merle et al., 1989; Platt et al., 1989; Ratschbacher et al., 1989).
Balanced cross-sections of the entire collisional crust beneath the Mont Blanc massif (Butler, 1983) won little favour (Platt, 1984), as a thick-skinned mechanism of basement faulting and ductile deformation at depth appear more appropriate than this thin thrust-sheet model. Anyway, Butler was probably the first to have figured the existence of first-order ruptures involving the Moho surface in the Alpine lithosphere.
Physical conditions of the high-P metamorphism and related P-T paths were estimated in different tectonic units of the Western Alps, locally integrated by fission track evidence on their cooling evolution, e.g.: i) Austroalpine outliers: Mt Emilius klippe (Dal Piaz et al., 1983) and Etirol-Levaz slice (Kienast, 1983), evolving from the Variscan granulite-facies lower crust to nearly Tethys floor, and then from subduction to final exhumation; ii) Sesia-Lanzo zone: kinematic and thermal modelling (Rubie, 1984; Hurford & Hunziker, 1985; Oberhänsli et al., 1985; Gillet et al., 1986; Koons et al., 1987; Pognante, 1989); iii) Zermatt-Saas, Monviso, Voltri and other eclogitic ophiolites: e.g., Baldelli et al., 1985; Barnicoat & Fry, 1986; Fry & Barnicoat, 1987; Kienast & Messiga, 1987; Martin & Kienast, 1987; Nisio et al., 1987; Philippot, 1988; Philippot & Kienast, 1989); iv) Monte Rosa, Gran Paradiso and Dora-Maira basement nappes (Compagnoni & Lombardo, 1974; Chopin, 1984; Dal Piaz & Lombardo, 1986; Ballèvre, 1988), where the eclogitic imprint was dated as Late Cretaceous by supposedly retentive Rb-Sr and 40Ar-39Ar ages (Chopin & Maluski, 1980; Monié, 1985; Monié & Chopin, 1991, and refs therein); v) contrasting metamorphic signatures within the Austroalpine-Penninic nappe stack (Kienast, 1983; Ballèvre et al., 1986; Goffé & Chopin, 1986; Gillet et al., 1986; Pognante et al., 1987). Systematic fission track analyses and mineral dating provided new temperature-time constraints for polyphase deformation and uplift patterns in various tectonic units throughout the Alps (Hurford et al., 1989; Hurford, 1991, and refs. therein). In particular, the Oligocene exhumation of the Sesia-Lanzo eclogitic complex (Dal Piaz et al., 1972; Compagnoni et al., 1977) was inferred also from fission track data showing that it cooled below 250-200°C by ca 33 Ma and below 100°C by ca 25 Ma (Hurford et al., 1991). The Late Oligocene-Early Miocene kinematics of the Periadriatic fault system (Bigi et al., 1990, 1993), coupled with backfolding and uplift of the Penninic inner zone, were quantitatively evaluated by Schmid et al. (1987, 1989).
Coesite was discovered first in the eclogitic continental crust of the Dora-Maira nappe (Chopin, 1984; Kienast et al., 1991), then in supra-ophiolitic metacherts of the Piedmont zone (Reinecke, 1991). This finding dramatically increased the depth to which these continental and relatively light (hydrated) oceanic units were dragged down into the subduction zone, and the extent of their upward trajectories, and so further difficulties were added to the debated problem of the exhumation mechanism.
Ballèvre et al. (1986) stressed the different tectono-thermal signature shown in the Aosta valley by two couples of continental-oceanic nappes, i.e. the lower Austroalpine outliers and Zermatt-Saas ophiolite, both eclogitic, and the overlying Dent Blanche-Mt Mary-Pillonet thrust system and Combin zone, both eclogite-free. The traditional European provenance of the Penninic units, as a whole, was maintained by Debelmas (1986), Gillet et al. (1986), Mattauer et al. (1987), confirming that the orogenic wave progressively involved more external domains of the colliding lower plate. Fry & Barnicoat (1987) envisaged subcreting eclogitic ophiolites to the overriding slab in a thermally mature subduction zone, and their preservation thanks to combining buoyant uplift and persistent refrigeration by the collisional underthrusting of cold crustal materials. In the meantime, the Lausanne team provided a systematic field survey in the southern Valais with innovative contributions on the stratigraphy and structural setting of the Piedmont zone and the Mid-Penninic Grand St Bernard system (Marthaler, 1984; Steck, 1984; Sartori, 1987; Escher, 1988); this impressive field work is synthetized in regional sections across the north-western Alps, from the core of the Simplon-Tessin window to the Dent Blanche nappe and the outer molasse (Escher et al., 1988).
An extensional west-dipping mylonitic fault between the Ruitor polymetamorphic basement and its Late Paleozoic metamorphic clastic cover within the Grand St Bernard system was thought to have exhumed the eclogitic and blueschist rocks from the subduction zone through an east-vergent, tangentially extrusive wedge (Caby & Kienast, 1989).
New petrologic estimates were given for basement, cover and ophiolitic units in the Central and Eastern Alps (e.g. Koller, 1985; Heinrich, 1986; Miller, 1986; Frank et al., 1987; Zimmermann & Franz, 1989; Koller & Höck, 1990; and refs. therein), whereas the Alpe Arami-Cima di Gagnone garnet-peridotite and associated eclogitic ophiolites and metarodingites (Adula-Cima Lunga nappe) corroborated their international fame thanks to work of Evans and Trommsdorff team. Recognition of clastic glaucophane and lawsonite in a Late Turonian flysch unit below the Austroalpine frontal thrust near the Swiss-Austrian boundary confirmed the timing of the eoalpine subduction, and provided conclusive evidence for an early exposure of subducted ophiolites (Winkler & Bernoulli, 1986; Winkler, 1988).
The volume “Geodynamics of the Eastern Alps”, edited by Flügel & Faupl (1987), synthetizes principal results of an Austrian national research project mainly addressed to the eoalpine orogenic history in the Austrian Alps. It includes a number of analytical contributions on the Eastern Alps and two interpretative models (Tollmann, 1987; Frank, 1987). Tollmann (1987) confirmed the traditional arrangement of facies distribution at the end of the geosynclinal stage, evolving from Permian-Triassic crustal attenuation to Jurassic opening of two oceanic gateways, coupled with gravitational sliding in the Nortern Calcareous Alps. These decollement cover nappes were restored between the upper-inner Austroalpine domain and the Southern Alps, covering a basement presently buried in the Alpine infrastructure. Frank (1987) proposed a different paleogeographic restoration of the Northern Calcareous Alps, allocated along the outer Austroalpine, north of the Silvretta-Oeztal basement.
Deep structure
Systematic seismic soundings and exploration drilling in the Po plain by the Italian national petroleum company (AGIP) provided a detailed and chronologically well-constrained picture of the subsurface frontal thrust systems in the Southern Alps and northern Apennine (Pieri & Groppi, 1981). Former reconstructions of the buried Alpine infrastructure were completely renewed by the French, Italian and Swiss national projects of deep seismic sounding across the Western and Central Alps (CROP-ECORS, NFP-20, CROP-Central Alps) and by the European Geotraverse. The meeting "Deep structure of the Alps", jointly organized by the French, Italian and Swiss Geological Societies and held in Paris in December 1988, documented how profoundly these seismic experiments innovated traditional views on the deep structure of the Alps. The meeting volume, edited by Roure, Heitzmann & Polino, was issued in 1990. The most innovative result of the CROP-ECORS experiment was the recognition of a principal rupture within the Moho discontinuity instead of a continuous downbulge, or a not actualistic double subduction, so that the role of Adria as leading lithospheric plate and of Europe as subducting lower lithospheric plate was firmly established (Thouvenot et al., 1990; Polino et al., 1990; Fig. 13).
Figure 13. Two Moho surfaces in the core of the Western Alps.
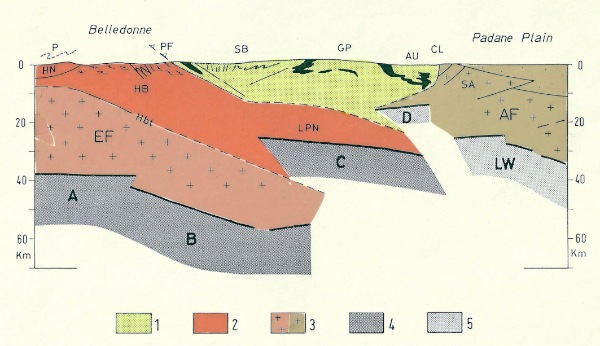
Geological interpretation of crustal image from wide angle and vertical seismic reflection experiments, CROP-ECORS transect (Polino et al., 1990): 1) remnants of Austroalpine-Penninic wedge, including Austroalpine (AU), ophiolitic (black), Gran Paradiso (GP), Grand St Bernard (SB) nappes; 2) deformed European passive margin: Helvetic-Ultraelvetic basement (HB) and cover nappes (HN), Lower Penninic nappe (LPN); 3) European foreland and its prolongation at depth (EF); 4) uplifted and back-thrusted crust of the Southern Alps (SA) and Adriatic foreland (AF). Canavese line (CL), Penninic frontal thrust (PF), Helvetic basal thrust (HbT), Prealpine nappes (P). Moho ruptures marked by European (A-B-C) and overlying Adriatic (LW) lithospheric mantle.
The seismic image well accounts also for a still ongoing indentation of the Southalpine prong into the back of the orogenic wedge, and for asymmetry of the collisional plate margin at a lithospheric scale. Similar Moho ruptures were found in the Swiss (NFP-20: Pfiffner et al., 1997) and Central Italian Alps (CROP-Alpi Centrali: Montrasio & Sciesa, 1994). The CROP Atlas, edited by Scrocca et al. (2003), includes the entire seismic data-set acquired during the CROP Project. Further geophysical experiments, their geological interpretation and overall reviews may be found in the special volumes on the European Geotraverse (Freemann et al., 1990; Pfiffner et al., 1990; Blundell et al., 1992, Capozzi & Castellarin, 1992, Schmid et al., 1994). I have drawn for this project the geological map of the Apennine and also that of the Italian part of the Alps, reported as made by another author.
Exhumation and wedge accretion
The problem of bringing deeply subducted continental and oceanic units to shallow crustal levels was thoroughly re-evaluated, whilst extensional tectonics became relevant as a tool to control or facilitate the exhuming processes (reviews in Platt, 1992; Ballèvre & Merle, 1993; Michard et al., 1993). These efforts produced a second-generation of kinematic models, some of them clearly influenced by exploration and modelling of modern accreting plate margins (details and refs. in Polino et al., 1990). The existence of a pre-collisional accretionary complex in the Alps, early figured by Roeder & Bögel (1978), was taken up by Treves (1984) who compared the so-called root zone (steep belt by Milnes, 1978; Milnes et al., 1981) to the inner sector of a modern tectonic wedge. A mechanism of upward flow (Pavlis & Bruhn, 1983) was thought to have assisted the uplift of subducted units, whereas a corner flow was favored by Winkler & Bernoulli (1986) in the Central Alps to generate the outer Penninic flysch prism and related ophiolitic detrital components.
An elegant underplating-wedge extension model under active convergence was proposed by Platt (1986, 1987) and then developed by Polino et al. (1990) with a different paleogeographic configuration of the intervening plates. The subduction-related high-P basement nappes were referred either to intra-oceanic microcontinents with European affinity (Platt, 1986), or to small fragments of the Adria active margin (Polino et al., 1990).
Extension during Eocene active convergence was suggested by Philippot (1988, 1990) in the axial-internal part of the Cottian Alps, where a stack of mafic eclogites (Monviso-Rocciavrè), coesite-bearing and eclogitic basement slices (Brossasco-Isasca complex, Dora-Maira; Kienast et al., 1990) and other lesser buried units are exposed. Continuous underthrusting of the colliding European lithosphere provided rapid uplift of the orogenic wedge, through concurrent development of synthetic and antythetic features and thrust-parallel low-angle normal faults. This was an alternative view to the classic twofold model of late-Alpine back-thrusting, a ductile to brittle deformation developed over the previous west-directed nappe stacking (e.g., Caby et al., 1978; Ballèvre et al., 1986). Neoalpine unroofing of the Lower Penninic nappes in the Simplon-Tessin area was assisted by the extensional low-angle Simplon fault (Mancktelow, 1985). A deep wedge was depicted by Hsü (1991), who still maintained the Briançonnais microcontinent and the Valais basin, even if no trace of the latter exists along the assumed Dora-Maira latitude, and referred the exhumation process to a persistent continent-ocean interaction, generating a tectonic melange at the plate boundary.
In the Eastern Alps, a pre-collisional sedimentary wedge of Eoalpine age and its collisional evolution were envisaged to explain the Matrei mixing zone and underlying Mesozoic calcschists at the tectonic boundary between the Tauern basement nappes and the Austroalpine leading edge (Frisch et al., 1987). On the basis of structural, strain and kinematic data, the Arosa zone at the Austroalpine/Penninic boundary was interpreted as an upper unit of the Cretaceous-Paleocene orogenic wedge, generated by concurrent offscraping of the Austroalpine hangingwall and accretion of oceanic material with greenschist to very low-grade imprint; a rather continuous change in tectonic transport from top-to-the west into top-to-the north across the Cretaceous-Tertiary boundary and successive accretion of Penninic units during outward propagation of deformation were clearly documented (Ring et al., 1988, 1989, 1990).
The generation of the Tauern window was adscribed to long-lived orogen-parallel extension of the Austroalpine orogenic lid, during active plate convergence, coupled with transversal shortening and doming of the Penninic basement nappes (Selverstone, 1985, 1988). The Tauern window is laterally limited by the antythetic Brenner and Katschberg normal faults (e.g., Fügenschuh et al., 1997; Genser & Neubauer 1989). This orogen-parallel extension and tectonic denudation developed in a late collisional stage and was accomodated by two-sided lateral extrusion of continental blocks (Ratschbacher et al., 1989, 1991). So, the traditional concept of alternating tensional and compressional episodes was ultimately abandoned, since active convergence actually persisted from the Cretaceous onwards (Dewey et al. 1989, and refs. therein).
Paleogeography and plate kinematics
The principal lithologies of modern oceans have been recognized in the Aosta valley-Valais area, internal Cottian Alps, Voltri Group and elsewhere throughout the Alps, showing mineral, isotope and geochemical evidence of ocean-floor igneous and hydrothermal activity (Mével et al., 1978; Dal Piaz et al., 1979, 1981; Bearth & Stern, 1979; Beccaluva et al., 1984; Pfeifer et al., 1989; Barnicoat & Cartwright, 1995; Cartwright & Barnicoat, 1999). In spite of this, the dominant stratigraphic setting can hardly be representative of a normal oceanic lithosphere because of: i) reduced overall thickness; ii) local absence of gabbros and/or basalts; iii) presence of abundant ophicarbonate breccias on top of serpentinized mantle peridotites, and of Jurassic metasediments with ultramafic detritus, or Mn-rich metacherts directly over serpentinites (Dal Piaz, 1969; Baldelli et al., 1983; Martin et al., 1994), indicating submarine mantle exposure before basalt extrusion. A sheeted dyke complex is missing everywhere, but some large fragments of serpentinized mantle peridotite that include hundreds and often thousands of iso-oriented rodingitic gabbro dykes may represent the remnants of a lithospheric-scale spreading center (e.g., Monte Rosso-Rocca di Verra and Mt Avic in the Aosta valley, Totalp and Oberhalbstein in Swiss Central Alps). Spalla et al. (1983) provided a detailed reconstruction of the folded contact between the Austroalpine Sesia-Lanzo inlier and the Lanzo ultramafic massif, and correlated the latter to the internal eclogitic unit (Zermatt-Saas) of the Piedmont zone.
Doubts were raised on the paleostructural meaning of the so-called ophiolitic sutures (e.g., Hawkesworth et al., 1975; Caron et al., 1987; Polino et al., 1990), since extensive transposition and out-of-sequence resetting were generated by selective offscraping of ophiolitic units from oceanic morpho-tectonic highs, rootless folding of imbricated nappes within the subduction complex, ductile exhumation and differential uplift by extensional detachments. Other doubts concerned the original allocation of the Austroalpine and Penninic nappes presently surrounded by ophiolitic units. The popular assumption of lithospheric microcontinents and narrow oceanic seaways can hardly explain the continuing subduction and rapid exhumation of high-P units without periodical obstructions, and the growing of the orogenic wedge itself, including basement nappes which seldom exceed 1-2 kilometres in thickness (Polino et al., 1990). Moreover, the huge amount of residual crust resulting from alleged collisional delamination of microcontinents must be stored in the orogenic roots or recycled into the mantle, but the expected anatectic melts are missing and it is unlikely that the mantle was energetic enough to recycle the crustal excess. Lastly, the minimum wavelength needed for multiple generation of rift-derived oceans alternating with microcontinents (400 km; Jolivet, 1995) implies an Alpine Tethys much wider than it probably was.
A renewed interest was directed towards the problems and global reconstruction of the Mediterranean area, i.e. motion of Africa relative to Europe, paleomagnetic and biostratigraphic constraints, palinspastic refinements of Tethys oceans, passive continental margins and intervening microcontinents, and the debated connection with the Atlantic spreading (e.g., Winterer & Bosellini, 1981; Frisch, 1981; Patriat et al., 1982; Savostin et al., 1986; Westphal et al., 1986; Abbate et al., 1988; Ziegler 1988; Dal Piaz & Polino, 1989; Dewey et al., 1989; Platt et al., 1989; Dercourt et al., 1990). Classic models on relative plate motion and generation of the Alpine belt by northward movement of Africa against Europe from the “mid” Cretaceous (90-80 Ma) onwards were confirmed (e.g., Olivet et al., 1984; Savostin et al., 1986; Dewey et al., 1989), or replaced with a dominantly NW- or WNW-directed convergence supported by kinematic indicators in major Alpine nappe systems (Baird & Dewey, 1986; Platt et al., 1989).
The Lower Jurassic-Cretaceous (190-90 Ma) sinistral movement between Africa and Europe was emphasized in actualistic reconstructions accounting for the opening of the Alpine-Apenninic (Piedmont-Ligurian) ocean, a northern segment of the Mid-Atlantic ridge displaced eastwards by the Newfoundland-Gibraltar transform system. Moreover, since some ophiolitic gabbros of the Alpine-Apenninic ranges yielded Late Triassic-Early Jurassic fission-track ages (Carpena & Caby, 1984), the onset of spreading in the Piedmont-Ligurian basin was thought to have developed independently from and before the central Atlantic opening and Africa-Europe strike-slip motion (e.g., Abbate et al., 1988). This is the case, however, only of pre-Jurassic gabbros that were generated and deformed during continental rifting (Lombardo & Pognante, 1982; Dal Piaz & Polino, 1989), opposite to Jurassic ridge-derived Mg- and Fe-Ti-gabbro bodies. These views anticipate simple-shear rifting reconstructions in which the early stage of ocean opening was supposedly generated by asymmetric extensional denudation of the subcontinental mantle and related pre-oceanic gabbros, as predicted by Elter (1972), postulated by Lemoine et al. (1987) and then corroborated by Vissers et al. (1991) and other authors. Such a model, however, is contrasted by some gabbros actually generated and deformed during continental rifting (Lombardo & Pognante, 1982; Dal Piaz & Polino, 1989). These views anticipate simple-shear rifting reconstructions in which the early stage of ocean opening was supposedly generated by asymmetric extensional denudation of the subcontinental mantle and related pre-oceanic gabbros, as postulated by Lemoine et al. (1987) and then corroborated by Vissers et al. (1991) and other authors.
The puzzle of western Tethys in the Alpine domain noticeably benefited from actualistic comparisons and detailed stratigraphic analyses in the Piedmont-Penninic ophiolites. As in the Ligurian nappes (Abbate et al., 1988), rather atypical ophiolitic associations with respect to the normal oceanic lithosphere were extensively recognized in the Alps: they consist of serpentinized mantle peridotite and/or discontinuos metagabbro bodies, directly covered by metamorphic ophiolitic sandstones, breccias or melange, in turn followed by tholeiitic metabasalts (locally absent), manganiferous quartzites (cherts) and other oceanic metasediments. Traces of a high-T oceanic metamorphism are scatterly preserved (Mevel et al., 1978), but a diffuse low-grade oceanic alteration is indirectly documented by geochemical and mineral data from eclogitic to greenschist facies metabasalts derived from protoliths enriched in Na and OH. Inferred paleostructural settings were thus compared with fracture zones and various types of topographic elevations in modern oceans (Lombardo & Pognante, 1982; Auzende et al., 1983; Beccaluva et al., 1984; Lagabrielle et al., 1984; Lemoine, 1984; Polino & Lemoine, 1984; Weissert & Bernoulli, 1985; Lagabrielle, 1987; Dal Piaz & Polino, 1989). The Piedmont-Ligurian ophiolites were genetically connnected to these paleostructures, thought to be selectively scraped off the oceanic lithosphere when they entered the subduction zone, were sliced and later exhumed through the orogenic wedge (see Polino et al., 1990, for details and refs.). Accordingly, the original width of the ocean cannot be inferred from the extent of Alpine ophiolitic remnants, as an unknown amount of oceanic lithosphere was definitively subducted. This casts doubts on the traditional view of a very small Piedmont ocean. Indeed, the existence of a relatively larger Alpine ocean is favored by the long-lasting (ca 40 Ma) subduction-induced thermal low recorded by the Austroalpine and Penninic units with high-P imprint (Polino et al., 1990; Dal Piaz, 1999). A minimum width of 300-400 km in the Piedmont-Ligurian ocean may be predicted if, in the Late Jurassic, Adria moved bodily with Africa (Abbate et al., 1988).
It has been generally accepted that the Ligurian-Piedmont basin in the Apennine and western Alpine domain represented a unique ocean of Mid-Late Jurassic age, as documented by radiolarian cherts of Callovian-Oxfordian age (De Wever et al., 1987, and refs. therein) and isotope dating (see Manatschal & Münterer, 2009, for review). Its NE-trend was depicted in most regional reconstructions (e.g., Dal Piaz, 1974; Winterer & Bosellini, 1981; Beccaluva et al., 1984; Lemoine et al., 1987; Abbate et al., 1988; Dal Piaz & Polino, 1989; and refs. therein). North of the French-Italian Alps, the Piedmont ocean was thought to be bounded by an east-west-trending shear zone, extending along the Swiss-Austrian Alps and Northern Carpathians: in the Late Jurassic the shear zone functioned as a left-lateral transform system, displacing a few short ridge segments eastwards and/or initiating some Penninic transtensional basins discontinuously floored by oceanic crust (Winterer & Bosellini, 1981; Beccaluva et al., 1984; Bernoulli & Weissert, 1985; Weissert & Bernoulli, 1985; Winkler & Bernoulli, 1986; Trümpy, 1988; Schmid et al., 1990). This reconstruction may replace the classic paired North- and South-Penninic oceanic gateways along the Swiss-Austrian Alps, a model that, however, continues to be supported (e.g., Tollmann, 1989; Dercourt et al., 1990; Thöni & Jagoutz, 1992, 1993). It does contrast also with the ENE-trending linear configuration of the Piedmon-Liguria ocean (Ziegler, 1988) and its orthogonal spreading (Dercourt et al., 1990). The northern shear zone could have functioned as a kinematic connection between spreading in the Piedmont-Ligurian ocean and consumption in the older Meliata-North Vardar ocean from the Mid-Late Jurassic onwards: the opening of the former was coeval with, and probably balanced by, the closure of the latter in the Carpathian domain.
In the western Southern Alps, extensional detachments, marked by high- to mid-grade mylonites, were recognized within the pre-Alpine lower crust of the Ivrea-Verbano zone, representing the deepest signature of the Mesozoic continental rifting now exposed in the Alpine realm (Hodges & Fountain, 1984; Brodie & Rutter, 1987; Handy & Zingg, 1991, and refs. therein). This record was the structural ground for new directions on the thermal-mechanical evolution of the Ivrea zone, based on simple-shear lithosphere attenuation and igneous underplating (details in the second part of the paper). In conclusion, the Mesozoic continental rifting was established in different structural settings of the Southern Alps (Bertotti et al., 1993, and refs. therein) and in other more fragmented segments of the Adria and Europe margins. Nevertheless, it can be historically interesting to recall that there was also a contrasting interpretation of the Middle-Triassic calc-alkaline magmatism in the Dolomites: alleged implications from the subduction-related geochemical affinity and local recognition of epidermic compressional features were believed to support the existence of a rapidly aborted Triassic orogeny also in the Southern Alps (Castellarin & Rossi, 1981; Bosellini et al., 1982).
Orogenic models
Contrasting paleostructural and kinematic models were elaborated by Hunziker et al. (1989) and Polino et al. (1990), starting from the intriguing Late Cretaceous age of the subduction metamorphism in the Monte Rosa and other inner Penninic nappes, and their traditional restoration in the distal continental margin of Europe. Indeed, as previously mentioned, an eoalpine age had been early envisaged also for the eclogitic imprint in the whole inner Penninic nappes (Dal Piaz et al., 1972), by extrapolation of Cretaceous-Paleocene K-Ar and Rb-Sr ages of white micas (90-53 Ma) regionally obtained in the eclogitic Sesia-Lanzo zone (Hunziker, 1974) and underlying Piedmont ophiolitic units (Bocquet et al., 1974). This hypothesis was corroborated by Cretaceous 40Ar-39Ar dating, systematically obtained on high-P micas from the Gran Paradiso (Chopin & Malusky, 1978, 1980), Monte Rosa (Chopin & Monié, 1984; Paquette et al., 1989) and Dora-Maira (Monié & Chopin, 1991) nappes. Interference patterns demonstrate that the high-P fabrics are overprinted by the Late Eocene-Early Oligocene (mesoalpine) greenschist to amphibolite facies metamorphism (Hunziker, 1974; Monié, 1985; Hunziker et al., 1992). In conclusion, the problem of excess argon was underestimated and the existence of a subduction metamorphism of eoalpine age wholly across the Austroalpine-Pennidic collisional zone appeared to be established. In this view, however, the alleged Late Cretaceous age of the eclogitic imprint in the European distal passive margin (upper-inner Penninic nappes) was incompatible with the evolution of the Ligurian-Piedmont ocean, which was still open at that time, as documented by deposition of trench sediments.
This inconsistency was tackled by Hunziker et al. (1989) and Polino et al. (1990) through alternative paleostructural scenarios, suggesting that the high-P Penninic nappes could have been wholly allocated in the frontal active margin and scraped off its underside by tectonic erosion, a mechanism favoured also by Hsü (1991). Tectonic sampling of continental fragments from the previously dismembered and then inverted Adria passive margin was supposedly generated by topographic highs (indenters) on the ocean floor when they entered the subduction gateway (Polino et al., 1990). This view avoided the problem of the Piedmont basin, but at the cost of an extreme resetting of classic paleogeography, as most units of the Penninic zone were removed from the European passive margin and allocated to the Adriatic one. A similar restoration was envisaged for the Monte Rosa domain also by Laubscher & Bernoulli (1982). Starting from this paleostructural setting, an even more complex tectonic mixing of continental and oceanic nappes by rootless folding and ductile shearing was assumed for generating the present structure of the collisional wedge.
Paired subduction zones inside a single ocean or of multiple oceanic branches were proposed by Radelli & Desmons (1987), Avigad et al. (1993), Michard et al. (1993). In particular, Avigad et al. (1993) again supported the classic provenance of the Dora Maira and other inner Penninic eclogitic nappes from the European passive margin, and supposed that these units were subducted beneath the Piedmont oceanic lithosphere, in turn consuming at the Adria margin, where a precollisional orogenic wedge (including the Sesia-Lanzo zone) was growing. As the Piedmont ocean was completely consumed, the subduction zone in the European side met the precollisional wedge at the opposite Adriatic side, generating an overthickened collisional wedge. From the Late Cretaceous onwards, extensional collapse and uplift of this gravitationally instable megasuture facilitated the Eocene collisional subduction of the Briançonnais domain which is recorded by a blueschist facies metamorphism. The Monte Rosa and Dora Maira nappes were restored in the European margin by Pfiffner (1992) and Avigad et al. (1993), whereas the Gran Paradiso nappe was allocated together with the Sesia-Lanzo zone along the Adriatic margin, in spite of the identical lithology, tectono-metamorphic features and structural position recorded by the Monte Rosa and Gran Paradiso basements.
The kinematic evolution of the Western Alps was tackled again by Ballèvre & Merle (1993) and Wheeler & Butler (1993), mainly dealing with extensional tectonics and exhumation of coesite-bearing and eclogite facies units from the subduction zone. Ballèvre & Merle (1993) envisaged the collisional stacking of Adriatic and Piedmont units over the European margin during the Lower-“mid” Cretaceous, followed by the activity of a late-eoalpine (90-60 Ma) crustal detachment, named Combin fault; this extensional event displaced the leading nappes toward the south-east, allowing the high-P units to be exhumed. Wheeler & Butler (1993) suggested the extensional displacement, to the south-east, of the Sesia-Lanzo basement with respect to the underlying ophiolitic Combin unit in the Ayas-Gressoney area (Aosta valley). It can be noted, however, that both units display the same polyphase imprint and an eventual unroofing detachment could better be located at a lower structural level, along the Combin/Zermatt-Saas tectonic contact which marks a first-rank metamorphic and chronological gap (Ballèvre & Merle, 1993; Dal Piaz, 1999; Dal Piaz et al., 2001; De Giusti et al., 2004). To sum up, most of Alpine Tethys reconstructions envisaged one or more microcontinents alternating with a number of oceanic channels (Piedmont and Valais ± Antrona ± Platta or Ligurian), merely inferred from the occurrence of three to four ophiolite-bearing structural levels within the orogenic wedge (e.g., Elter & Pertusati, 1973; Pasquarè, 1975; Trümpy, 1980; Platt, 1986; Mattauer et al., 1987; Pfiffner, 1992; Ring, 1992). These reconstructions were essentially inferred from the implicit assumption that, during the orogeny, the paleogeographic domains were converted into a pile of nappes, the superposition of which wholly replicates their originally lateral juxtaposition as in Argand’s fold-nappe model.
Conceptual models of rifting and continental margins were critically discussed by Stampfli et al. (1991). Two rifting types of Mesozoic Tethys (Neotethys) were recognized: i) a Greece-Turkey system genetically connected eastwards to the Permian Tethys, with ocean spreading delayed until Middle Triassic; ii) an Alpine system, genetically linked to the opening of the central Atlantic and characterized by a Late Triassic transtensive phase, an Early-Middle Liassic break-away phase, and a Middle-Late Jurassic ocean spreading. In addition, Stampfli (1993) elaborated an innovative reconstruction of the Briançonnais (Grand St Bernard) domain. It was envisaged as an exotic terrane, formerly belonging to the European continental margin and then displaced eastwards during the Late Jurassic-“mid” Cretaceous drifting of Iberia, a process that in turn allowed the Cretaceous opening of the Valais ocean and the coeval closure of the Piedmont ocean. The resulting oblique collision between the Briançonnais microcontinent and the Adria margin supposedly generated the Cretaceous high-P metamorphism, whereas the westward thrusting of the Briançonnais over the European margin was the early Tertiary result of consumption of the Valais basin. As discussed in Dal Piaz (1999), this creative model does not account, however, for the absence of significant ophiolitic remnants along the Penninic/Helvetic boundary in the French-Italian Alps, where Stampfli’s southward extension of the Valais ocean should have been sutured.
In the Central Alps, the polyphase tectono-metamorphic evolution of the Shams and Tambo nappes and some N-Penninic ophiolitic units were investigated (Schmid et al., 1990; Schreurs, 1993; Baudin & Marquer, 1993). Whilst previous strike-slip-dominated reconstructions of the Piedmont-Ligurian (S-Penninic) ocean in the central Alps were confirmed (e.g., Beccaluva et al., 1984; Weissert & Bernoulli, 1985), opening of the Valais (N-Penninic) basin and its westward narrowing were noticeably innovated, suggesting that it developed within a left-lateral mega-shear zone. In particular, the Valais trough was extended to the Tauern domain, according to current Austrian views (e.g., Ring, 1992; Thöni & Jagoutz, 1993), the Suretta nappe was coupled with Tambo, forming an intra-oceanic eastwards extension of the Briançonnais high, characterized by high-P relics (Baudin & Marquer, 1993), and the eclogitic Adula nappe (Heinrich, 1986) was allocated in a very external position, near the Helvetic domain. The timing of the eclogitic metamorphism in the Adula nappe (Hunziker et al., 1989) and the mechanism of its exhumation are enigmatic in this paleogeographic scenario, both related to the Oligocene-Neogene accretion of the Helvetic foreland. An Oligocene subduction of the N-Penninic ocean was supposedly corroborated by a zircon age (28.5 Ma) from the garnet-peridotite/eclogite association of the Adula-Cima Lunga nappe (Gebauer et al., 1992). The geological meaning of this age is doubtful, since the eclogitic stage was followed by the Eocene-Lower Oligocene Barrovian overprint (Frey et al., 1974), the Oligocene intrusion of the Bergell pluton and its erosion during the deposition of Upper Oligocene Gonfolite. Recent Sm-Nd dating of the same mafic-ultramafic association in the Adula-Cima Lunga nappe yielded more consistent mineral ages at around 40 Ma, rightly thought to be cooling ages that probably approximate the eclogite facies climax (Becker, 1993).
The geodynamic evolution of Pennine nappe stack in the eastern Central Alps was refined through thermobarometric and kinematic data (Ring, 1992), whilst interaction between deformation and metamorphic processes (Selverstone, 1993), thermal history and heterogeneous uplift (Reddy et al., 1993) were estimated in the Tauern window. Updated reviews of pre-Mesozoic geology, radiometric dating, tectono-thermal evolution and kinematics in the Eastern Alps, particularly concerning the Austroalpine nappe system, was reported in the ALCAPA Field Guide (Neubauer, 1992).
The intrusion, thermal metamorphism and ductile deformation of the Oligocene Bergell pluton developed during contraction of the Central Alps, before the Neogene transpression of Periadriatic fault system, as accurately investigated by Rosemberg et al. (1994) and Berger et al. (1996), developing a topic previously described by Wenk (1973) and Conforto-Galli et al. (1988). The tectono-magmatic evolution of some sheeted intrusions (lamellae) along a proto-Giudicarie fault was described by Martin et al. (1993), as evidence of Late Oligocene ductile deformations during the strike-slip movement of the Tonale-Giudicarie fault system.
Extended reviews of the first twenty-five years of plate tectonics in the Alps and elsewhere are reported in the proceedings of the VIII Summer School, University of Siena, edited by Giorgio Ranalli (1995). In the last decade of the Twentieth Century the essential headlines of plate tectonics have not been significantly modified their influence on Alpine geology, continuing to provide a solid framework for refinement of classic interpretations or provocative views based on further geological, geophysical and petrological advances. Among them, overall plate kinematics was renewed envisaging a global westward drift of the lithosphere coupled with relative eastward counterflow of the asthenosphere (Doglioni, 1990). The model and its development well account for differing features of present and past opposite-vergent subduction zones, related foredeeps, accretionary wedges and thrust belts, particularly regarding the Alpine and Apennine domains (Doglioni, 1992, 1994; Doglioni et al., 1997, 1999).
Geochronology: innovation and inferences
The Alpine overprint in the Eastern Austroalpine is documented by post-Variscan cover metasediments and had been dated as eoalpine (100-70 Ma) also in the crystalline basement (Thöni, 1999, and refs therein). Its ranges from very low-grade (Silvretta and northern Oetztal) to greenschist, amphibolite (staurolite-kyanite, southern Oetztal), and local eclogite facies conditions in mafic rocks (Frey et al., 1999). Far to the east, in the Koralpe-Saualpe (Koriden) country - the type locality of eclogite (Godard, 2001) - two main basement units with contrasting metamorphic features were recognized and re-interpreted through innovative dating. The former, also occurring in the Pohorje ranges, consists of kyanite-rich paragneisses with abundant bodies of fresch to amphibolitised eclogite from basalt and gabbro protoliths of Permian age (Thöni & Jagoutz, 1992, 1993; Miller & Thöni, 1997; Thöni & Miller, 1996), manganiferous quartzites, marbles, calc-silicate rocks and pegmatites; its subduction metamorphism is eoalpine, effacing most of previous low-P (andalusite) features. The latter and overlying complex (micaschist group), including the ophiolite-bearing Plankogel unit, is characterized by an eoalpine medium-grade overprint.
In the Western Alps the subduction metamorphism was formerly referred to a single orogenic event of Cretaceous-Paleocene age (90-53 Ma), called eoalpine, as supposedly established by K-Ar, 40Ar-39Ar and minor Rb-Sr data (Hunziker, 1974; Bocquet et al., 1974; Hunziker et al. 1992). As previously seen, this dating strongly influenced the paleostructural reconstructions of Alpine Tethys and its orogenic contraction (Dal Piaz et al. 1972; Dal Piaz, 1994, 1995; Avigad et al., 1993; Michard et al., 1993, 1996). In the secon half of 1990s, the age of subduction metamorphism was re-considered thanks to new dating on retentive systems (Gebauer, 1999, and Thöni, 1999, for reviews) and recognition of excess Argon in micas previously dated as eoalpine (Arnaud & Kelley 1995; Reddy et al. 1996; Ruffet et al. 1997). Hence, the eoalpine high-P event was consistently restricted to the Austroalpine by Late Cretaceous ages obtained in the Sesia-Lanzo zone (Ramsbotham et al. 1994; Venturini, 1995; Inger et al. 1996; Duchêne et al. 1997; Rubatto et al., 1999). Similar ages clustering around 74 Ma were obtained also in the Austroalpine Pillonet klippe by coherent 40Ar-39Ar and Rb-Sr data on phengitic micas coexisting with glaucophane-crossite (Cortiana et al., 1998).
Conversely, the subduction metamorphism in the classic area of the Zermatt-Saas nappe (Valais-northern Aosta valley) was rejuvenated to the Early-Middle Eocene, based on dating of mafic eclogites from Zermatt (Bowtell et al. 1994, Sm-Nd: 50 ± 18 Ma) and St Jacques (Mayer et al. 1999, Sm-Nd: 49 ± 4 Ma, Rb-Sr: 46 Ma), and UHP metachert-eclogite couple of Cignana lake (Rubatto et al. 1998, U-Pb: 44.5 ± 2.3, 43.9 ± 0.9 Ma; Amato et al. 1999, Sm-Nd: 40.6 ± 2.6 Ma). Similar ages were obtained in the Monviso ophiolite (Monié & Philippot, 1989; Duchêne et al., 1997; Cliff et al., 1998; Rubatto et al., 1998), and later in the Mt Avic massif, the southern extension of the Zermatt-Saas nappe south of the Aosta-Ranzola fault system (Dal Piaz et al., 2001; Beltrando et al., 2009). Furtermore, the Cretaceous 40Ar-39Ar ages obtained in the Monte Rosa and Gran Paradiso basement (Chopin & Maluski, 1980; Chopin & Monié, 1984; Monié, 1985) were supposed to be influenced by excess Argon, like those in the coesite-bearing Dora-Maira nappe (Arnaud & Kelley, 1995) which underwnt ultrahigh-P metamorphism in the Late Eocene (Tilton et al., 1989, 1991; Gebauer et al., 1995). The expected Eocene age of the eclogitic metamorphism in the Gran Paradiso and Monte Rosa nappes will be established ten years later, by Meffan et al. (2004), Lapen et al. (2007) and Gabudianu Radulescu et al. (2009), like in the Adula-Cima Lunga nappe (Becker, 1993: 44-35 Ma).
To sum up, an Eocene age has been documented for the subduction metamorphism in the Penninic continental and ophiolitic units, by extrapolation of available data throughout the Alps (e.g. Blankenburg & Davies, 1995; Froitzheim & Manatschal, 1996; Michard et al., 1996; Schmid et al., 1996, 1997). Even if the Eocene ages obtained in the Piedmont zone concern only the Zermatt-Saas nappe and similar units, the main chronological obstacle to the classic restoration of the Penninic continental nappes in respect with the Piedmont basin was finally overcame.
In this view, a new reconstruction of the Western Austroalpine and Piedmont units and their restoration back to the initial configuratin of Mesozoic Tethys were conceived by Ballèvre et al. (1986), corroborated by Cortiana et al. (1998) and refined by Dal Piaz (1999), Dal Piaz et al. (2001), Bistacchi et al. (2001), focusing on the multiple alternances of Austroalpine and Piedmont units in the structural depression of the Aosta valley and their significance. This reconstruction is based on the present geometry of the nappe pile, new isotope chronology of the Late Cretaceous-Eocene subduction metamorphism, and the existence of extensional allochthons instead of lithospheric microcontinents (Fig. 14). The starting point was an innovative subdivision of the Austroalpine-Piedmont nappe stack inferred from the correlation of the intra-ophiolitic northern Austroalpine outliers (Etirol-Levaz, Grun, Chatillon-St Vincent) with the southern Austroalpine outliers (Mt Emilius, Glacier-Rafray, Tour Ponton, Santanel, Verres), all eclogitic and located respectively north (hangingwall) and south (footwall) of the north-dipping Aosta-Ranzola normal fault (Fig. 14). This does mean that the overlying Combin zone could formerly extend southwards over the Mt Emilius klippe, but clearly below the Sesia-Lanzo frontal thrust. In this view, the Austroalpine outliers (Dent Blanche nappe s.l.) and Piedmont ophiolitic units can be subdivided into two groups of continental-oceanic nappes characterized by contrasting P-T-time trajectories, as follows (Dal Piaz et al., 2001):
1) Eclogite-free upper group - It consists of the Dent Blanche-Mt Mary-Pillonet thrust system and the underlying Combin zone, both displaying a relatively high-P imprint which is dated as Late Cretaceous (74-73 Ma) in the Pillonet klippe by concordant Rb-Sr and Ar-Ar ages on white micas, coexisting with sodic amphiboles (Cortiana et al., 1998). The age of the Combin blueschist mineral relics is still unknown, even if a Late Cretaceous and/or Paleocene age can not be excluded since this ophiolitic nappe acted as a tectonic sole of the accreting Dent Blanche-Mt Mary-Pillonet thrust system.
Figure 14. Tectono-metamorphic setting of the Western Austroalpine-Penninic wedge in the Aosta valley and surrounding areas.
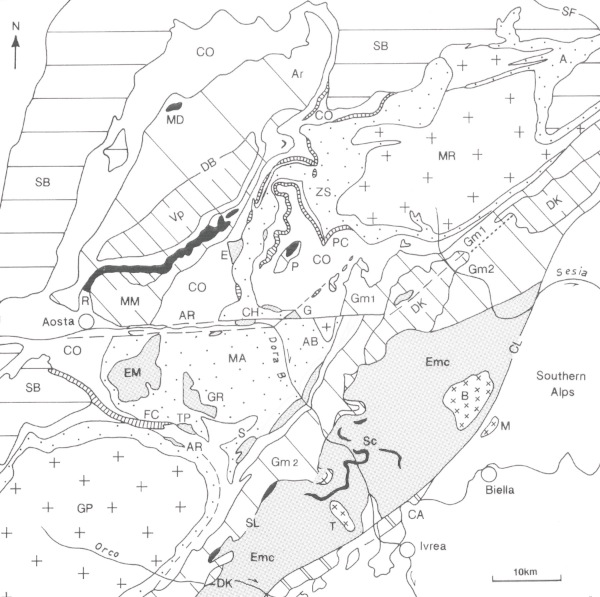
Austroalpine: 1) Upper outliers (non eclogitic): Dent Blanche (DB)-Mt Mary (MM)-Pillonet (P) thrust system; 2) Lower outliers (eclogitic): Acque Rosse (AR), Chatillon (CH), Etirol-Levaz (E), Grun (G), Mt Emilius (EM), Glacier-Rafray (GR), Santanel-Verres (S), Tour Ponton (T); 3) Sesia-Lanzo zone (SL). Lithology: Valpelline-2nd Diorite-kinzigitic series (Vp-Dk), lower crust; Arolla series (Ar), Gneiss minuti complex (Gm1: non eclogitic, Gm2: eclogitic relics); Eclogitic micaschist complex (Emc); Mesozoic metasedimentary units: Roisan zone (R), Sesia-Lanzo (Sc). Ophiolitic Piedmont zone: 1) Relatively high-P (non eclogitic) Combin zone (CO), including Pancherot-Cime Bianche (PCB), Frilihorn and Cogne (FC) Permian-Mesozoic decollement cover units of continental affinity; 2) Eclogitic Zermatt-Saas zone (ZS) extending to the Mt Avic massif (MA); Antrona ophiolite (A). Penninic continental nappes: Monte Rosa (MR), Arcesa-Brusson (AB), Gran Paradiso (GP) inner-upper Penninic nappe system; mid-Penninic Grand St Bernard composite nappe system (SB). Canavese zone and Canavese tectonic line (CA), Aosta-Ranzola fault system (AR), Simplon normal fault (SF). Slightly modified from Dal Piaz (1999).
2) Eclogitic lower group – It consists of the Mt Emilius (Fig. 15) and other lower Austroalpine eclogitic outliers and underlying or interbedded units of the Zermatt-Saas nappe. Their typical eclogitic imprint yielded Early-Mid Eocene ages (50-44 Ma), opposite to the Late Cretaceous eclogitic micaschist complex in the inner Sesia-Lanzo zone. The original source of the Dent Blanche-Mt Mary-Pillonet thrust system and the Sesia-Lanzo zone may be a set of extensional allochthons probably juxtaposed to the Adriatic margin. Indeed, the oceanic seaway envisaged by Stampfli (1993) between the Dent Blanche-Sesia and Canavese domains is virtually possible but not supported by robust surface evidence (discussion in follows). The ophiolitic units of the Combin zone were located in the internal edge of the Piedmont ocean and then accreted, from the beginning of subduction onwards, below and in front of the Dent Blanche-Mt Mary-Pillonet thrust system. In contrast, the Zermatt-Saas ophiolite and the eclogitic lower Austroalpine outliers derived from one or more intraoceanic allochthons both originated far to the north-west and entered the subduction zone 30 Ma later than the upper group of continental-oceanic nappes. A relatively wide and now lost sector of the Piedmont-Ligurian ocean was probably interposed between the Combin and Zermatt-Saas oceanic domains, filling the gap in the chronological record.
Figure 15. Mt Emilius klippe.
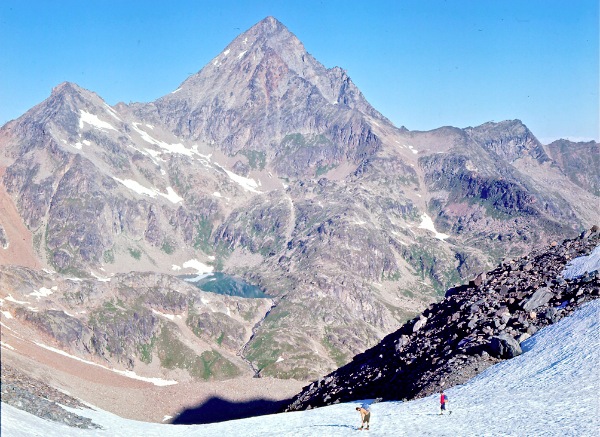
South-eastern face of the Lower Austroalpine Mt Emilius outlier from Col di Leppe: Eclogitic micaschist complex with Eocene high-P imprint, derived from a kinzitite-type suite and minor granitoids (Dal Piaz et al., 1983, 2001).
At the beginning of the Third Millenium
The last decade is characterized by the increasing number of papers, the development of international teams and the flowering of a new generation of gifted and very active young researchers, also positively inclined to the field work. This decade is too cloose for any historical overview of Alpine Geosciences. Therefore, I shall dwell only on some innovating or conflictual points, integrated by personal comments mainly concerning the continental and oceanic units with high-P imprint, their protoliths and the overall architecture and paleogeographic scenarios of the Alps.
Tectono-metamorphic evolution and geochronology
A new map at one million scale showing the metamorphic structure of the Alps has been compiled by an international working group and edited by Oberhänsli et al. (2004). It focuses on the polyphase Alpine metamorphism, disregarding older metamorphic events. The map is based on the spatial distribution of metamorphic facies, like former metamorphic maps did (Niggli et al., 1978; Frey et al., 1999), but is integrated by information on the type and grade of the Alpine metamorphism, interpreted as a geodynamic marker. The various metamorphic peak conditions are dated in a schematic inset which represents three chronological groups of P-dominated (subduction-related) metamorphism (110-90, 89-60 and 59-35 Ma) and two groups of T-dominated (syn- to post-collisional) metamoprhism (110-60 and 59-0 Ma, cooling ages). Fifteen metamorphic facies are chromatically distinguished and assembled in three groups which show the regional distribution of tectonic units dominated by subduction, continental collision and high-T exhumation features, regardless of their Cretaceous or Tertiary age. Summing up, the structural-metamorphic map simply shows the geodynamic evolution of the Alps that evolved through two subsequent orogenies (Cretaceous and Cenozoic).
Western Alps - Beginning with the ophiolitic Piedmont zone, a number of consistent U-Pb, Sm-Nd, Rb-Sr and 40Ar-39Ar dating confirmed the Mid-Eocene age of the eclogitic and ultrahigh-P metamorphism in the Zermatt-Saas nappe, within the 47-42 Ma time span, both north of the Aosta-Ranzola fault (Rubatto et al., 1998; Lapen et al., 2003; Gouzu et al., 2006; Herwartz et al., 2008) and south of it, from the Mt Avic massif to the Urtier valley (Rubatto et al., 1998; Dal Piaz et al., 2001; Beltrando et al., 2009a), as well as in similar occurrences of the Cottian Alps (Lombardo et al., 2002; Rubatto & Hermann, 2003) and Voltri massif (Rubatto & Scambelluri, 2003; Federico et al., 2007). The ultrahigh-P unit of Lago di Cignana was mapped in detail (Forster et al., 2004) and became the object of further field and laboratory work (Lapen et al., 2003; Gouzu et al., 2006; Müller & Compagnoni, 2009; Skora et al., 2009). In particular, Groppo et al. (2009) confirmed that the Lago di Cignana and Zermatt-Saas units display identical metamorphic evolutions, being differentiated only by the presence or absence of coesite, and restricting the pressure difference to less than 0.3 GPa. Diamonds long searched and never found within the garnet peridotite-eclogite bodies of the Central and Eastern Alps (do you remember the recurrent debate between Green and Trommsdorff ?) have been discovered in manganiferous garnet-rich nodules from the coesite-bearing Lago di Cignana unit (Selverstone et al., 2010). It has to be emphasized that these are the first diamonds known in the Alps, the first from oceanic metasediments, and the lowest-T occurrence (≤600°C) yet reported from the ultrahigh-P rocks.
The predicted Eocene age of the relict eclogitic imprint in the underlying upper-inner Penninic basement nappes of the Graian and Pennine Alps (e.g. Dal Piaz, 1999), already assessed in the Brossasco-Isasca unit of the Dora-Maira nappe, was finally established, yielding 43 ± 0.5 Ma in the Gran Paradiso silvery micaschists (Meffan et al., 2004: apatite-phengite Rb-Sr microsampling), and 42.6 ± 0.6 Ma (Lapen et al., 2007: U-Pb on rutile, carbonate and quartz-white mica fractions from quartz-carbonate veins within the mafic eclogite) in an eclogitic boudin from the polymetamorphic Furgg Zone in the southern Monte Rosa (Dal Piaz, 2001b; Fig. 16). New petrological estimates and SHRIMP dating on the same silvery micaschists of the Gran Paradiso nappe have been recently presented by Gabudianu Radulescu et al. (2009), showing that the metamorphic peak (2.7 GPa /515–600°C) of these metasomatic and mylonitic granitoids could have been developed near the ultrahigh-P field and at the Eocene-Oligocene boundary, based on U-Th dating of allanite (33.7±1.6 Ma) and prograde monazite (37.4 ± 0.9 Ma). Doubts on the geological meaning of the younger age arise from the Arcesa-Brusson window, southern Monte Rosa nappe and their Zermatt-Saas envelope, since these basements with eclogitic relics and greenschist facies regional fabrics were cooled below 250°C at 33 Ma (Gran Paradiso, Hurford A. J. & Hunziker, 1989) and rigid enough to be cut by lamprophyre dykes, listvenitic faults and gold-bearing quartz vein, yielding 32-29 Ma and 32-30 Ma, respectively (Dal Piaz et al., 1979; Venturelli et al., 1984; Pettcke et al., 1999; Bistacchi et al., 2001). The Arcesa-Brusson window is open in the footwall of the Aosta-Ranzola normal fault, of Oligocene age, between the Monte Rosa and Gran Paradiso massifs, and display the same basement units of these nappes (Bigi et al., 1990). Therefore, I like better dating and interpretation of Meffan et al. (2004). Otherwise, the Gran Paradiso and Monte Rosa nappes followed very similar, but diachronous P-T-paths.
Figure 16. Eclogites of Monte Rosa nappe.
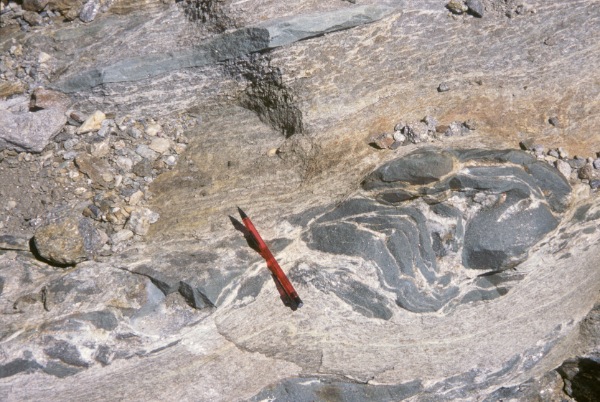
Mafic boudins with Eocene eclogitic imprint (42.6 ± 0.6 Ma; Lapen et al., 2007) inside the polymetamorphic Furgg Zone, derived from pre-Variscan continental flood basalt; Indren glacier, southern Monte Rosa (Dal Piaz, 2001b).
Anyway, the supposed robust eoalpine Rb-Sr and 40Ar-39Ar ages of the Monte Rosa and Gran Paradiso internal nappes were ultimately dismissed, together with their role in costraining the age of the ocean closure, syn-collysional subduction of the European distal margin, and alternative kinematic models (e.g. Polino et al., 1990). Indeed, the nearly identical P-T evolution of the Gran Paradiso, Arcesa-Brusson, Monte Rosa and Zermatt-Saas nappes (Antrona included) suggests that these high-P continental and oceanic units shared a common tectono-metamorphic history during a crucial phase of the Alpine orogeny (for alternative views see Froitzheim, 1999; Schmid et al., 2004; Pleuger et al., 2006). Their close time-space association probably played a key role in exhumation and preservation of the eclogite-facies ophiolites through buoyancy-driven uplift of the underlying continental nappes (Lapen et al., 2007), and with the significant help of highly hydrated fragments of oceanic lithosphere (Poli & Schmidt, 1995; Angiboust & Agard, 2010), mainly represented by serpentinized mantle peridotites (Breithorn-Verra, Avic, Voltri; Bigi et al., 1990).
Detailed information on contraction and extension of the Austroalpine-Penninic collisional wedge based on isotope dating and kinematic analysis has been given by Reddy et al. (2003), dealing with a significant transect across the Gressoney, Ayas and Tournanche valleys, from the Sesia-Lanzo Zone to the Dent Blanche nappe, through the composite ophiolitic Piedmont zone. An initial phase of accretion and NW-directed thrusting at ca. 60 Ma is inferred from two white mica Rb-Sr ages of Bec di Nana basal milonite, the northern edge of the Pillonet klippe (Dal Piaz, 1976), where better preserved high-Si micas in equilibrium with blue amphiboles yielded Rb-Sr and 40Ar-30Ar ages of 74 Ma (Cortiana et al., 1998), a more reliable age for thrusting of the upper Austroalpine outliers. Other Rb-Sr white mica dates (48-36 Ma) from different kinematic domains (Reddy et al., 2003) document the age of deformation and fabric formation under greenschist facies conditions within the ophiolitic Combin zone, or cooling in the Zermatt-Saas nappe. Contrasting top-to-NW and top-to-SE kinematics are recognized: the latter deformation is related to crustal extension and is consistent with exhumation of underlying eclogitic units, whereas 40Ar-39Ar data, if not influenced by excess or loss Argon, reflect the cooling evolution of eclogitic and greenschist facies units between 40 and 32 Ma (Reddy et al., 2003).
Eastern Austroalpine - A bimodal tectono-metamorphic model was envisaged also by Thöni (2006), based on an updated review of petrological estimates and isotope dating of eclogitic units in the Austroalpine and Penninic nappes of the Eastern Alps. Mafic eclogites and high-P metapelites along the inner Austroalpine in the Texel (1.3 GPa/520–600 °C), Schober (1.8 GPa/≤690 °C) and Saualpe-Koralpe-Pohorje units (2.2–≤2.7 GPa/≤750 °C), display a similar structural position and almost identical Cretaceous ages (90-85 Ma) on Sm–Nd, Lu–Hf, U–Pb and Rb–Sr systems, pointing for a common subduction and exhumation history. This “eoalpine high-pressure belt” is supposed to have been derived from the distal passive margin of a Meliata back-arc basin and, further west, from the Austroalpine crust along an intracontinental subduction zone during the Cretaceous Apulia-Europe plate convergence, with mean exhumation rates of 5–≤10 km/Ma between 90 and 85 Ma (Thöni, 2006). Otherwise, the underlying Penninic nappes display, in the Tauern window, an eclogitic imprint (2.4 GPa/≤630 °C) and a rapid exhumation/cooling dated as Eocene-Early Oligocene (<45–31 Ma) through amphibole and phengite 40Ar–39Ar and Rb–Sr multimineral isochron ages. The high-P event is related to the subduction of the former European continental margin after the closure of the Penninic ocean, and its accretion at the base of the Mesozoic ophiolitic units and capping Austroalpine nappe stack (Thöni, 2006). Further precise dating of eclogitic gabbros from the Kupplerbrunn–Prickler Halt eclogite type-locality (Haüy, 1822, in Godard, 2001) and other Saualpe localities can be found in Thöni et al. (2008).
In spite of this, the classic concept of older eclogites in the Eastern Austroalpine was not abandoned in the Eastern Austroalpine (Magetti et al., 1987; Miller & Thöni, 1995; Godard et al., 1996; Martin et al., 1998, 2009; Tumiati et al., 2003), as the existence of Variscan eclogites from MORB-type protoliths was confirmed and corroborated in the crystalline basement of central Ötztal (2.7 GPa/730 °C; mean Sm–Nd age: 347 ± 9 Ma), eastern Silvretta (ca 350 Ma), and Ulten units (336 ± 4 Ma), all thought to be representative of the North-Apulian (Austroalpine) paleostructural domain (Thöni, 2006). Earlier assumptions of an eclogitic event in the Eastern Austroalpine of Caledonian (Purtscheller & Sassi, 1975) or Neoroterozoic age (Manby et al., 1988) was ultimately invalidated.
Pohorje massif - In the last decade, the eclogite and peridotite bodies long known inside the Slovenian Pohorje massif (Hinterlechner-Ravnik, 1982; Hinterlechner-Ravnik et al., 1991a-b) at the easternmost edge of the Alps (Bigi et al., 1990) became the subject of renewed interest (Sassi et al., 2004; Janak et al., 2006; Thöni et al., 2008). The mafic rocks had been interpreted as relics of oceanic crust with a pre-Alpine, possibly Caledonian, high-P metamorphism (Hinterlechner-Ravnik et al., 1991b). According to Sassi et al. (2004), lenses of kyanite- and quartz-rich eclogites derived from gabbroid cumulates and more fractionated protoliths are embedded within amphibolite-facies quartz-feldspathic gneisses, both characterized by a N-Morb type signature. Eclogitic P–T conditions were estimated 1.8–2.5 Gpa/630–700°C, consistently with the absence of coesite. By comparison with the similar Koralpe and Saualpe basement units, the high-P metamorphism of the Pohorje mafic rocks was referred to subduction of oceanic crust during the Cretaceous collision (ca 100 Ma) between the European and Apulian plates. The garnet-peridotites of the Pohorje massif were quoted as evidence of ultrahigh-P conditions (Janak et al. (2006): these rocks, which probably derive from a depleted mantle source, display four main stages of crystallization, evolving from a prograde path (spinel I → garnet) to a decompressional path (garnet → spinel II → amphibole-serpentine). This evolution is similar to that described in the Ulten unit (Godard et al., 1996; Martin et al., 2009), where similar Sm-Nd ages (330 Ma) suggest that all rock types shared a common history since the incorporation of the peridotite in the crust, and constrain the garnet-facies of peridotites to crustal subduction at the end of the Variscan orogeny (Tumiati et al., 2003). Peak estimates by for garnet-peridotites are 900°C/4 Gpa (Janak et al., 2006), and the inferred geodinamic interpretation suggests that the ultrahigh-P metamorphism is a result of deep subduction of continental crust which scraped mantle slices off the lithospheric upper plate. The principal unroofing stages developed from the Upper Cretaceous to the Miocene formation of an extensional core complex. The eoalpine age of the high-P imprint in the Pohorje massif was documented by Thöni et al. (2008), where a low-Mg eclogite provided a garnet–whole-rock Lu–Hf age of 93 Ma, similar to those reported by the same authors for various eclogites of Saualpe. In conclusion, Thöni et al. (2008) suggest that garnet growth in the Saualpe–Pohorje high-P units developed between ca 95–94 Ma and ca 90–88 Ma, probably at the final pressure peak. Rapid exhumation of the high-P units was related to the collision of the northern part of the Apulian plate with parts of the Austroalpine microplate, which developed after the Jurassic closure of the Permian-Triassic Meliata back-arc basin.
Tauern window – The physical conditions of eclogites occurring in some Penninic units are confirmed by recent calibrations of garnet-omphacite-phengite-kyanite-quartz mineral assemblages, giving values of about 2.5 GPa/630°C, which approach the stability field of coesite (Hoschek, 2007). The kyanite-eclogites include a strongly retrogressed jadeite-gneiss, derived from siliciclastic deposits and consisting of garnet-quartz-white micas, with kyanite and Na-pyroxene only as inclusion in garnet (Miller et al., 2007). Peak metamorphic conditions of the relict primary assemblage are 2.4–2.0GPa/640°C. U–Pb zircon dating yielded only prealpine ages, supporting the existence of three igneous events in the source area of zircons, at 466 ± 2 Ma, 437 ± 2 Ma and 288 ± 9 Ma.
Paleostructural restoration and Tethys reconstruction
A new tectonic map of the entire Alps at about 1:2,500,000 scale has been compiled by Schmid et al. (2004), starting from the Structural Model of Italy (Bigi et al., 1990, 1992). The orogenic evolution of the Alps is discussed and based on the integration of surface and subsurface structural data, contrasting tectono-metamorphic events and paleogeographic restoration of cover and basement units back to Mesozoic Tethys. The concept that the Alps are a bimodal orogenic belt (Froitzheim et al., 1996) is strenghtened and developed. The first orogeny of Cretaceous age (eoalpine) is recorded only in the Eastern Alps and is followed by a second orogenic cycle of Tertiary age, with a Late Cretaceous phase of extension and exhumation between them (Froitzheim et al. 1994; Ratschbacher et al., 1989). The former orogeny is due to the subduction-related closure of the Triassic Meliata ocean, supposedly tailing into the Apulia (Adria) continental margin, the latter to the classic closure of the Jurassic Alpine Tethys between Apulia and Europe. For the sake of clarity, it may be recalled that the classic term Tethys has been recently used in various ways (e.g., Stampfli et al., 2001a-b): i) Paleotethys, a mainly Paleozoic ocean restored north of the Cimmerian continent; ii) Neotethys, a late Paleozoic-Mesozoic ocean allocated south of this continent, including the Meliata, Vardar and other Triassic-Jurassic seaways, developing independently from the central Atlantic; iii) Alpine Tethys or Westewrn Tethys, the Middle Jurassic ocean including the Ligurian-Piedmont (South-Penninic) and Valais (North-Penninic) basins, and genetically linked to the opening of the central Atlantic. The original extension of the Meliata ocean into the future Alpine domain of the Adriatic plate is not documented by direct evidence, being limited to a few slices of serpentinite and Mesozoic basinal sediments in the eastern edge of the Northern Calcareous Alps. Schmid et al. (2004) have tentatively attributed the crystalline nappes of the Koralpe-Wölz high-P system (Miller & Thöni, 1997; Hoinkes et al., 1999; Schuster et al., 2001; Schuster, 2003) to Neothetys and its distal passive margin, being aware that this reconstruction is speculative. The Mesozoic cover of parts of this nappe system was detached before the eoalpine high-P metamorphism and final closure of the Meliata Ocean, like the Pancherot-Cime Bianche cover unit later did during the western Alpine contraction (Dal Piaz, 1999). In spite of the absence of a robust ophiolitic suture, the existence of a Triassic ocean and its consumption is indirectly inferred (Schmid et al., 2004) as a geodynamic engine accounting for the generation of a pre-Gosau orogenic wedge and related eclogitic (subduction) imprint to high-T Barrovian (collisional) metamorphism of eoalpine age (Early-Mid Cretaceous, Thöni, 1999, 2006).
A similar reconstruction has been suggested by Dal Piaz et al. (2003), pointing for the different strutural position (early established by Argand) between the Eastern Austroalpine tectonic system and the underlying Western Austroalpine (Adria-derived Dent Blanche and Sesia-Lanzo nappes), as well as for their different tectono-metamorphic evolution. As clearly pointed out by Schmid et al. (2004), some points of their stimulating work may be controversial and deserve to be developed:
1) The existence in the Eastern Alps of two independent orogenies (Cretaceous vs. Tertiary), clearly separated by a syn-Gosau extensional phase, is a milestone of the memoir of Schmid et al. (2004). However, extension in the Eastern Austroalpine seems rather an exuming-accretion process in the leading edge during active plate convergence (Ratschbacher et al., 1989). In any case, while the Eastern Alps enjoyed this break in shortening deformation, the Western Alps were involved into lithospheric contraction with top-to-the-west tectonic transport (Dal Piaz & Sacchi, 1969), and the Late Cretaceous subduction metamorphism recorded in the Sesia-Lanzo Zone and Pillonet klippe. Therefore, a transition (partial overlapping) rather than a clear-cut separation seems to be the better solution, as also suggested by Trümpy (1992).
2) The continental basement and cover units inside the Tauern window are wholly referred to the Sub-Penninic nappes (distal European margin), as the Ossola-Tessin dome, whereas the overlying Glocknerdecke (the fixist Obere Schieferhülle) becomes a North-Lower Penninic (Valais) unit, according to Froitzheim et al. (1996), who equated this ophiolitic nappe with the Bündnerschiefer of the Engadine window. The Glocknerdecke is capped in turn by the Matrai shear zone (Bigi et al., 1990) interpreted as a S-Penninic (Piedmont) unit (Schmid et al., 2004): it marks the Penninic/Austroalpine boundary and consists of tectonic mixing of ophiolitic calcschists, Triassic marbles and Austroalpine basement slices (Gb Dal Piaz, 1934; Frisch et al., 1987; Kurz et al., 1998 a-b). The absence of continental nappes between the North and S-Penninic units postulated by Schmid et al. (2004) implicitly supports the existence of a unique Penninic ocean. This paleogeographic reconstruction has been criticized by Kurz (2005) and confirmed by Schmid et al. (2005) with additional comments. In my opinion the problem is still open, since: i) the Glockner nappe display a close lithological and metamorphic affinity with the ophiolitic units of the Combin zone (South-Penninic); ii) the “non-eclogitic Sub-Penninic basement nappes” of the Tauern window includes bodies of fresch to retrogressed ecogites (Bianchi, 1934; Cortiana et al., 2004; Thöni, 2006); iii) the blueschist and eclogite facies metamorphism in various oceanic and continental units of the Tauern window is dated as Eocene (Ratschbacher et al., 2004; Glodny et al., 2005; Thöni, 2006; Kurz et al., 2008), like in the inner-upper Penninic Monte Rosa-Gran Paradiso nappes and overlying Zermatt-Saas ophiolite; iv) the garnet-kyanite-chloritoid quartzschists from the Eclogite Zone racall the eclogitic mylonites of the Monte Rosa nappe; v) in the central Lower Penninic zone only the Adula nappe displays a lithology and eclogitic imprint (Fig.17) similar to the Monte Rosa nappe, and its sub-penninic allocation is valid only from a geometrical point of view; vi) the Late Jurassic Hochstegen marble points for the extension of the Briançonnais facies into the Tauern window. For these reasons, I am inclined to like better the solution that it was preferred for these nappes when I compiled the Alpine area of the Structural Model of Italy (Bigi et al., 1990).
3) Again about the Valais (N-Penninic) domain, the Antrona ophiolite is traditionally and better referred to the Zermatt-Saas nappe, instead of to the N-Penninic, due to their closely similar lithological, geochemical and metamorphic features (Beccaluva et al., 1984; Colombi & Pfeifer, 1986; Pfeifer et al., 1989; Dal Piaz, 1999), even if the Zermatt-Saas metabasalts display a much greater compositional variety and often a spilitic composition (pillow lavas) in respect with the Antona unit, the latter providing chemical evidence of fast spreading (Pfeifer et al., 1989). The present position of Antrona at the base of the Monte Rosa fold-nappe is referred to the Alpine contraction and is incorporated into the Zermatt-Saas domain (Dal Piaz et al., 1972; Polino et al., 1990). A different view is proposed by Froitzheim (2001).
Figure 17. Eclogites of Adula nappe.
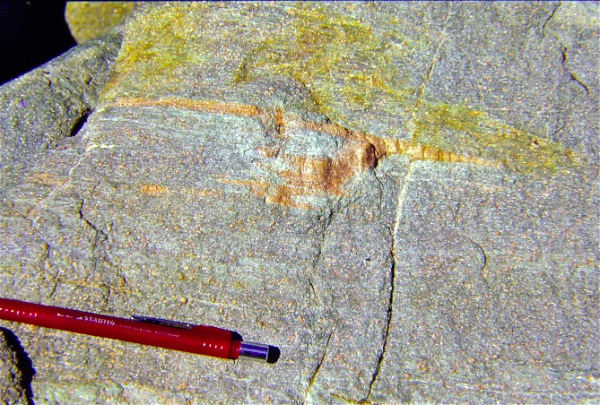
Eclogite with isoclinal folding within high-P micaschists of Adula nappe, Fanella pass.
4) Traditionally coeval with the Piedmont-Ligurian ocean, the N-Penninic (Valais) basin has been rejuvenated to the Early Cretaceous, thanks to stratigraphic evidence in the central Alps (Froitzheim & Manatschal, 1996) and the kinematic connection with the Bay of Biscay (Stampfli, 1993). In addition, Stampfli (1993) postulated the extension of the Valais oceanic basin wholly along the French-Italian Alps, in spite of the absence of ophiolitic traces south of Moutiers, where the N-Penninic units disappear along the Briançonnais/Helvetic-Dauphinois boundary (e.g. Dal Piaz, 1974, 1999; Sturani, 1975; Compagnoni et al., 1977). Starting from Stampfli’s assumption, the Mid-Penninic Grand St Bernard tectonic system became a Briançonnais exotic terrane (extensional ribbon continent), that developed since the Cretaceous birth of the Valais basin and concurrent contraction of the Piedmont ocean (Stampfli, 1993, 1994, Stampfli & Borel, 2002; Stampfli et al., 2002). The Briançonnais terrane also encompasses the Inner-Upper Penninic (prePiedmontese) Monte Rosa, Gran Paradiso and Dora-Maira nappes (Elter, 1960, 1972; Dal Piaz et al., 1972; Dal Piaz, 1974; Sturani, 1975). The exotic terrane is believed to wedge out between the Engadine and Tauern windows and therefore it is no more present among the core nappes of the Eastern Alps (Trümpy, 1988; Schmid et al., 2004, Figs 1 and 2). As figured in Bigi et al. (1990) and maintained by Dal Piaz et al. (2003), from the beginning of the Jurassic spreading the Monte Rosa-Gran Paradiso domain belonged to the ocean-facing distal edge of the European passive continental margin, since in the Eocene it was subducted below the Adriatic leading margin together with the Piedmont oceanic lithosphere and just after the Middle Eocene basin closure.
5) The Western Austroalpine Dent Blanche and Sesia-Lanzo tectonic system (Dal Piaz et al., 1972; Compagnoni et al., 1975, 1977) is renamed by Schmid et al. (2004) either Lower Austroalpine nappes (Plate 1) or Margna-Sesia fragment, according to Froitzheim et al. (1996). The former term rightly emphasizes the unquestionable low-standing position of these units in respect with the entire Eastern Austroalpine, a tenet firmly established by Argand through his famous figure in which the Eastern Austroalpine nappe stack is projected over the six Penninic fold-nappes, Dent Blanche included, but this name is misleading due to the existence of Lower Austroalpine units in the Eastern Alps, which are characterized by contrasting structural position, lithology and metamorphic features. Therefore, in the Western Alps it is preferred the neutral name Western Austroalpine, also because, as previously seen, this is a double nappe system grouping upper and lower outliers with a different and diachronous subduction metamorphism (Dal Piaz, 1976, 1999; Ballevre et al., 1986; Dal Piaz et al., 2001; Beltrando et al. 2010b).
6) The term Margna-Sesia fragment, recently rediscovered as Cervinia (Pleuger et al., 2007), recalls the continental unit early envisaged by Compagnoni et al., 1975, 1977), and the intraoceanic restoration of the Margna nappe and Sesia-Lanzo Zone supported by Froitzheim et al. (1996), even if the present structural settings of these nappes is different. Indeed, these units are separated by a Jurassic right-lateral shear zone (Dal Piaz, 1999) and while the Margna nappe is sandwiched between two distinct ophiolitic units (Pasquarè, 1975; Compagnoni et al., 1975, 1977), the Sesia-Lanzo is thrusted over the entire Piedmont zone and on its internal side is laterally juxtaposed to the Ivrea-Verbano lower crust (Southern Alps), through the ophiolite-free Canavese zone (Ferrando et al., 2004) and related bounding faults (Bigi et al., 1990). As previously seen, a similar position is assumed for the non-eclogitic Dent Blanche-Mt Mary-Pillonet thrust system (upper Austroalpine outliers), whereas the eclogitic Mt Emilius and other lower Austroalpine outliers (Glacier-Rafray, Chatillon, Grun. Etirol-Levaz, Santanel, Verres) are presently located at the boundary between the Combin and Zermatt-Saas nappe, and within the latter. Back to the paleogeographic puzzle, a further oceanic gap has been postulated between the Sesia-Lanzo domain and the Southern Alps also by (Froitzheim et al., 1994, 1996;; Froitzheim & Manatschal, 1996). This is virtually possible, since a subducted ocean may not leave superficial traces, but as a matter of fact it is not supported by an unquestionable ophiolitic suite, so that the classic allocation of the Dent Blanche-Sesia (extensional allochthons) along the proximal Adriatic margin (Dal Piaz, 1999) is considered more reliable. Their Adriatic affinity is documented by similar crustal protoliths (Franchi et al., 1908; Novarese, 1931), and this was pointed out also by Trümpy (1992), grouping the Dent Blanche, Simme and Margna nappes as Ultrapennine units, with Triassic facies belts continuing into the western Southern Alps. An intraoceanic source is more likely in the case of the Mt Emilius and other lower Austroalpine outliers that entered the subduction zone and acquired their eclogitic imprint together the Zermatt-Saas nappe, 30 Ma after the Sesia-Lanzo zone and upper Austroalpine outliers acquired their subduction metamorphism (Dal Piaz, 1999; Dal Piaz et al., 2001; Beltrando et al., 2010b).
7) The classic and popular term “Penninic”, long consolidated in Alpine literature since Argand’s work, cannot be avoided even if it was and stil is used in different misleading ways. Indeed, as mentioned by Schmid et al. (2004), the term “Penninic nappes” generally indicates tectonic units coming from multiple continental and oceanic domains (European margin, Valais ocean, Briançonnais terrane, Piedmont-Ligurian ocean), and it was maintained by these authors in a reduced form, i.e. excluding the Sub-Penninic units coming from the European margin, north-west of the Valais basin. Alternatively, the Penninic term can be restricted to the continental nappes derived from the Jurassic European passive margin and eventually related ribbon continents (Dal Piaz, 1974, 1999), all characterized by an eclogitic or blueschist facies imprint of Eocene age, opposite to the Helvetic-Ultrahelvetic units that were accreted to the collisional belt later and only when the subduction-related thermal low had been definitively re-equilibrated. In addition, the precise provenance of the Piedmont-Ligurian ophiolitic units with respect to the unknown Jurassic spreading center can not be unequivocally defined, as they may be derived from the oceanic side of the Adriatic, European, or both plates, hence the uncertainty between a Penninic or non-Penninic affiliation.
8) A flake-tectonic model of the Eastern Alps has been proposed by Schmid et al. (2004) along the Transalp transect and the Austroalpine nappe stack east of the Tauern window, suggesting a provocative northward subduction of the Apulian plate. The former profile is a provisional alternative interpretation of the Transalp deep seismic experiment, whilst the latter is inferred only from tomographic information on mantle slab (Lippisch, 2002).
Western Austroalpine - Unforeseen Eocene ages on high-Si white micas (Rb-Sr, Ar-Ar: 49-42 Ma; Dal Piaz et al., 2001; Beltrando et al., 2009a) and zircon (U-Pb: 47.3 ± 1.3 Ma; Beltrando et al., 2009b, 2010b) were obtained for the eclogitic imprint recorded by the lower Austroalpine outliers (Mt Emilius, Glacier-Rafray, Tour Ponton, Acque Rosse, Eirol-Levaz), previously believed to be essentially coeval with that in the inner Sesia-Lanzo zone (85-69 Ma). These basement slivers are intimately associated to fragments of oceanic lithosphere which display the same and coheval eclogitic imprint, suggesting that their former juxtaposition probably goes back to the rifting stage (Dal Piaz, 1999; Dal Piaz et al., 2001, 2004; Beltrando et al., 2010a-b). All this points for the existence of a peculiar oceanic lithosphere located near the passive continental margin, and characterized by oceanic crust locally roofed by some thin extensional allochthons that did not alter the essential features (sea-floor morphostructure, overall thickness) and properties (thermal setting, negative buojancy, rheology) of the Piedmont-Ligurian lithosphere at the end of spreading and thus favorable for deep subduction (Dal Piaz, 1999). By comparison with the Galicia-Iberia margin (Boilot et al., 1995; Manatschal & Bernoulli, 1999; Peron-Pinvidic et al., 2007), this peculiar crust has been reported as ocean-continent transition, a very popular term (Manatschal et al., 2003; Ferrando et al., 2004; Manatschal, 2004; Bernoulli & Jenkins, 2009; Beltrando et al., 1910a-b). Anyway, this anomalous crustal zone is a proximal part of the Zermatt-Saas external sector of the Piedmont ocean, far from the internal Combin zone, where extensional allochthons of continental basement are lacking. Clues of ocean-continental transition are reported from the Gets decollement nappe which includes ophiolites of Jurassic age and continental crust elements (Bill et al., 1997, 2000).
My opinion on the debated paleostructural reconstruction of the western Alpine Tethys (e.g., Froitzheim, 2001, Rosenbaum & Lister, 2005, for reviews) is based on these constraints and assumptions (Dal Piaz et al., 1972, 2001; Bocquet, 1974; Compagnoni et al., 1977; Cortiana et al., 1998; Dal Piaz, 1999): i) stratigraphic and radiometric data indicate that the onset of subduction and orogenic contraction propagated from the Sesia-Lanzo inlier to the Grand St Bernard domain, through the consuming Piedmonte ocean; ii) Late Cretaceous age of the blueschist relics in the Pillonet klippe and other upper Austroalpine outliers; iii) concurrent development of the eclogitic imprint in the lower Austroalpine outliers and associated Zermatt-Saas ophiolites; iv) a delay of 30-25 Ma between the two recorded events; v) the occurrence of the Combin zone as a tectonic sole of the upper Austroalpine outliers, displaying similar blueschist relics of potential latest Cretaceous and/or Paleocene-Early Eocene age; vi) disappearence at depth of large portions of normal to anomalous oceanic crust; vii) close lithostratigraphic and metamorphic features of the prealpine Sesia-Lanzo and Dent Blanche protholiths with the Southalpine continental crust; viii) a certain pre-piedmontese and briançonnais affinity of the Permian-Mesozoic Pancherot-Cime Bianche decollement unit, clearly different from the Roisan zone; this exotic unit is presently interbedded within the oceanic units of the Combin zone (e.g. tectonic inset in Bucher et al., 2003-04), and probably it had been detached from the cover-free lower Austroalpine outliers at incoming subduction. Evaluating pros and cons, I maintain the Austroalpine (Adriatic) affinity of the Sesia-Lanzo zone, the Dent Blanche-M.Mary-Pillonet upper Austroalpine outliers and their Mesozoic metasedimentary cover, regardless of the presence or absence of a thrue ophiolitic suite as evidence of an oceanic channel along the Canavese fault system. By contrast, the Mt Emilius, Glacier-Rafray, Etirol-Levaz and other lower Austroalpine eclogitic outliers probably derived from one or more extensional allochtons trapped within the Combin/Zermatt-Saas boundary zone and/or in the latter oceanic domain, thus assuming a proximal Penninic (European) affiliation and partly going back to Argand’s views. Please note that this does not mean that I agree with an European origin of the Sesia-Lanzo inlier, a concept early alleged by Aubouin et al. (1977), Fudral & Deville (1986), Mattauer et al. (1987).
Figure 18. Recumbent backfolding in southern Monte Rosa massi.
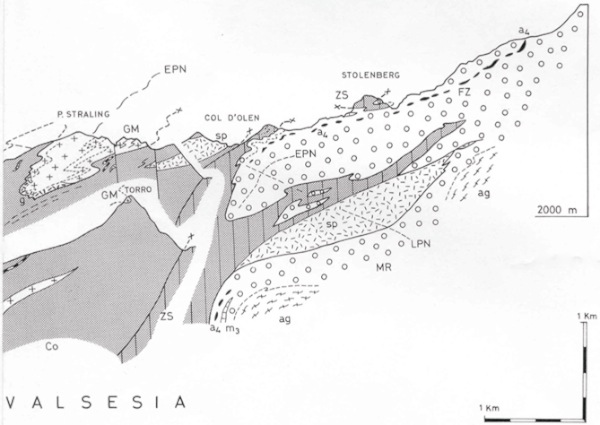
Section of the collisional suture along the ridge between the Lys and Sesia valleys (Gosso et al., 1979). From top to bottom: 1) Sesia-Lanzo zone: Gneiss minuti (GM) and minor mylonitic gabbros (g); 2) Piedmont zone: Combin (Co) and Zermatt-Saas (ZS) units: major serpentinite bodies (sp); 3) Monte Rosa nappe: dominant garnet-micaschists (MR) with lenses of eclogite-amphibolite (a4) and rare beds of old marble (m3); augengneiss (ag) from Late Paleozoic granite. Monte Rosa and Zermatt-Saas nappes are folded together, independently from the later tectonic contact between the two ophiolitic units (named Combin fault by Ballevre & Merle, 1993). Early (EPN) and late (LPN) postnappe deformations.
Valais basin - Herwartz et al. (2008) and Pleuger et al. (2005, 2007) have enucleated from the Zermatt-Saas nappe a supposedly independent and younger ophiolitic unit - called Balma - that in the southern side of the Monte Rosa massif is involved within the recumbent back-folds of the Monte Rosa basement nappe (Gosso et al., 1979; Dal Piaz, 1992). This reconstruction is based on U-Pb SHRIMP ages of ca 93 Ma yielded by zircon cores in a mafic eclogite collected near the Colle delle Pisse station of the Alagna-Passo dei Salati cable-car. On this ground, the eclogitic Balma ophiolite has been re-interpreted as a sliver of Late Cretaceous oceanic crust, extending from Alagna to the Stolemberg infrastructure, through the Bors valley, and believed to be representative of the N-Penninic (Valais) oceanic basin, like the Antrona unit and the ophiolitic slices inside the Swiss Furgg Zone. I do not agree with this interpretation, because of numerous cons in face of a doubtful meaning of zircon dating, e.g.: i) the Zermatt-Saas and Balma units in the Alagna-Gressoney area display the same lithostratigraphic features, structural setting, mineral composition, polyphase metamorphic evolution, deformation history, isotope dating and geochemical affinity (Dal Piaz et al., 1981; Beccaluva et al., 1984; Pfeifer et al., 1989; Herwartz et al., 2008); ii) the alleged two units are physically continuous even if boudinaged or sheared within tight synformal structures, including mylonitic serpentinites and chlorite-actinolithe schists (e.g., Stolenberg, Colle delle Pisse, Malfatta, Punta Perazzi; Fig. 18). Following previous reconstructions (Froitzheim & Manatschal, 1996; Froitzheim et al., 1996; Froitzheim, 2001), Pleuger et al. (2007) have proposed the intra-Piedmontese to oblique opening of a Cretaceous Valais basin, the sub-penninic allocation of the Monte Rosa domain, the origin of the Sesia-Dent Blanche system from a continental fragment (labelled Cervinia) within the Alpine Tethyan ocean, and the suture of the ophiolitic Combin (Tsatè) unit between the Sesia-Lanzo and the Southern Alps, that I can not share at all.
An oceanic channel along the internal side of the Sesia-Lanzo fragment may be eventually conceived as the original country of the Ligurian-type (obducted) ophiolitic materials embodied within the sedimentary Gets nappe (e.g. Dal Piaz, 1999; Fig.19), that however was not dragged down into the subduction zone instead of the Combin zone. Ophiolitic gabbros from the Gets nappe yielded two identical U-Pb zircon ages of 166 ± 1 Ma and a 40Ar/39Ar plateau age on amphibole of 165.9 ± 2 Ma (Bill et al., 1997), like in the Piedmon-Ligurian zone. Tectonic denudation of litospheric mantle and associated gabbros at the floor of the Jurassic Piedmont ocean is documented in the Lanzo massif (Lagabrielle et al., 1989; Pelletier & Müntener, 2006) and in the huge Mt Avic massif (Tartarotti et al., 1998; Dal Piaz et al., 2010), a correlation that is strengthened by similar Eocene ages of the high-P metamorphism in the Lanzo (Rubatto et al., 2008) and Avic massif (Dal Piaz et al., 2001). Trace of tectonic mantle denudation in the Canavese area (discussion in Ferrando et al., 2004) may be eventually envisaged within the scarce and scattered tectonic slices of serpentinized lherzolite and minor altered gabbro, long ago known within the Canavese region (Novarese, 1929; Elter et al., 1966; Sturani, 1975), without supporting the existence of a true ophiolitic suite as envisaged in some modern works.
Figure 19. Western AlpineTethys.
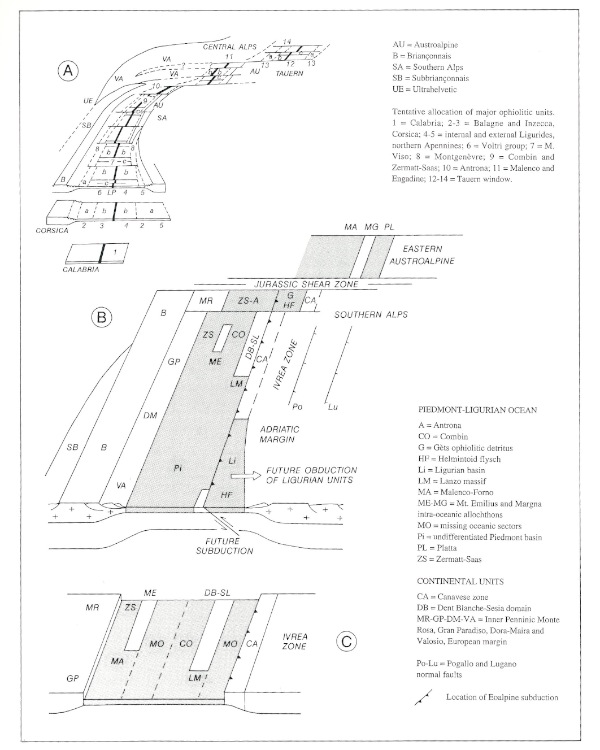
Reconstruction of western Alpine Tethys in Late Jurassic (Dal Piaz, 1999). A) Overall restoration of Piedmont-Ligurian basin (Beccaluva et al., 1984): oceanic crust with T-MORB (a) to N-MORB (b) affinity, fracture zones (c) with gabbro and/or serpentinite bodies on ocean floor. B) Details of central and western Alpine Tethys, showing Mt Emilius and Margna extensional allochthons dispersed within Mesozoic ocean. Note the Ligurian and Gets oceanic lithosphere inside the future upper plate. C) Alternative reconstruction assuming an intraoceanic origin also for Dent Blanche-Sesia domain. Not to scale.
While the existence of the Cretaceous Valais ocean (Balma units) south of the the Monte Rosa domain was suggested and emphasized, the classic Versoyen ophiolite (Martin et al., 1994; Cannic et al., 1996; Bousquet et al., 2002) was losing their Mesozoic age and paleostructural role (Beltrando et al., 2007; Masson et al., 2008). Indeed, the mafic rocks of the Versoyen unit, long envisaged as derived from the Mesozoic oceanic floor of the Valais basin, recently provided Permian (Beltrando et al., 2007: 272 ± 2, 267 ± 1) or Visean (Masson et al., 2008: 337.0 ± 4.1) U-Pb zircon ages, resulting a Variscan ophiolitic suture (Schärer et al., 2000; Masson et al., 2008) or a post-Variscan igneous suite emplaced into the continental crust during a process of lithospheric stretching before the break-up of Pangea (Beltrando et al., 2007, 2010a). In any case, whatever the age would be, the existence of a Cretaceous ocean in the external Penninic zone of the Western Alps becomes very improbable (Masson et al., 2008), with inferences of the Briançonnais terrane itself.
Focusing on north-western Tethys, an innovative scenario of the Cretaceous tectonic setting has been proposed by Beltrando et al. (2007), starting from their work on the Versoyen, literature data on the Central Alps (Florinet & Froitzheim, 1994; Liati et al., 2003), and previous paleostructural reconstructions given by Stampfli et al. (2002) and Pleuger et al. (2006). From south to north, a large Alpine Tethys and the Iberia-Briançonnais microplate can be recognized, followed by the Chiavenna-Balma oceanic branches (ca 95-90 Ma), the Valais intracontinental-oceanic basin and, between them, the Dora-Maira and Adula ribbon continent. This paleostructural configuration is the result of the left-lateral megashear zone operating between Europe and Iberia at ca 110-90 Ma.
Mesozoic and older global reconstructions - Global tectonics of the perimediterranean orogenic belts was refined by Stampfli & Hochard (2009), suggesting that the East-Mediterranean basin belonged to the larger Neotethyan ocean that opened during the Permian. The Mesozoic Apulia domain grouped the autochthonous units of Greece and SW Turkey, and the unitary Adria-Apulia microplate since the Early Jurassic. A new post-Variscan continental fit for the western Tethys was presented, and inferred from the relationships between the Adriatic, Apulian and Iberian plates, as well as a detailed palinspastic model of the Alpine domain from the Late Triassic to the Miocene (Stampfli & Hochard, 2009). Thereby the geodynamic scenario for the Alpine orogeny is refined, emphasizing the contrasting evolution of the Eastern Alps-Carpathians belt with respect to the Western Alps. Generation of the former orogenic segment was related to a northward roll-back of the Maliac–Meliata slab and displacement of the Meliata suture and Austroalpine collisional belt into the eastern Alpine domain, whereas the Western Alps were characterized by the changing African plate boundary and the interaction between the Iberian–Briançonnais plate and the Austroalpine accretionary wedge (Stampfli & Hochard, 2009). The complex correlation of tectonic units within the Alps-Carpathians-Dinarides system is discussed by Schmid et al. (2008) and presented through a map and crustal-scale sections that provide a tridimensional view of the present structural setting and the overprinting effects of multiple deformations on these orogens.
Plate tectonic models of Paleozoic and older continental fit and geodynamic events, from spreading to subduction and continental collision, have been elaborated at a global scale by various authors (e.g. Stampfli & Borel, 2002; Stampfli et al., 2002; Von Raumer & Stampfli, 2008), also strenghtening Irving’s configuration of Pangea B in the Early Permian, and its transformation to Pangea B before the Late Permian (Muttoni et al., 2003, 2009), or reconstructing the evolution of Adria and wandering of the Austroalpine and Southalpine basement units presently located in the Alps (Spiess et al., 2010).
Adamello batholith and Periadriatic magmatism
As the Tertiary magmatism along the Periadriatic igneous belt from the Aosta Valley to the Pannonian basin is concerned (Bigi et al., 1990) (Fig. 20), recent works essentially concentrated on the structural features, emplacement mechanism and rock analogue experiments, and also confirmed previous dating of intrusive bodies: see the updated review given by Rosemberg (2004), together with discussion on the problem of magma ascent and shear zones. Focusing on the composite Adamello batholith and its internal subdivision in several plutons (Bianchi et al., 1970; Callegari & Gb. Dal Piaz, 1973; Callegari et al., 1998), it can be mentioned that the intrusion age had been previously established for the Re di Castello southern pluton (U-Pb: 42-39 Ma; Hansmann & Oberli, 1991), whereas the great body of Rb-Sr and K-Ar mica ages (Del Moro et al., 1985 a-b) well documented only the younging cooling history from the southern (42-38 Ma) to the central and northern plutons (30-29 Ma; discussion and complete refs. in Brack et al., 2008). Innovating data were given for the intrusion age of the entire batholith by Mayer et al. (2003): U-Pb age determinations on single zircon (CRPG-Nancy) carried-out for the central and northern plutons document the existence of three main phases of zircon growth, statistically recognised at abouth 42, 37 and 31 Ma. The two older phases are mostly recorded in zircon cores, while the younger one are more frequent at the rims, regardless of zircon morphology and cathodeluminescence microstructures. The age of 42 Ma is well represented in the Corno-Alto trondhjemite (thus coeval with the Re di Castello pluton), but also in the zircon cores from Marser gabbro, Central Adamello tonalite and other northern plutons. The most frequent zircon age of 37 Ma is widespread in Central Adamello tonalites, and is often found in all other intrusive bodies. The younger ages at zircon rims (31 Ma) commonly occur (not exclusively) in the border plutons of Val d'Avio, Val di Genova and Nambrone. The presence of these three chronological clusters in several plutons suggests that zircons had been resided, episodically or continuously, in contact with a Zr-oversaturated magma over a long time-period. This also imply that the generation of the Adamello batholith was a long-lasting process involving an internal recycling of magmatic components formed in different times in a common magma chamber (Mayer et al., 2003). Superheated friction-induced melts were carefully analysed in zoned pseudotachylytes occurring within the Adamello tonalites, and compared with laboratory experiments and earthquake simulations (Di Toro et al., 2004, 1911; Pennacchioni et al., 2006).
Figure 20. Periadriatic magmatism.
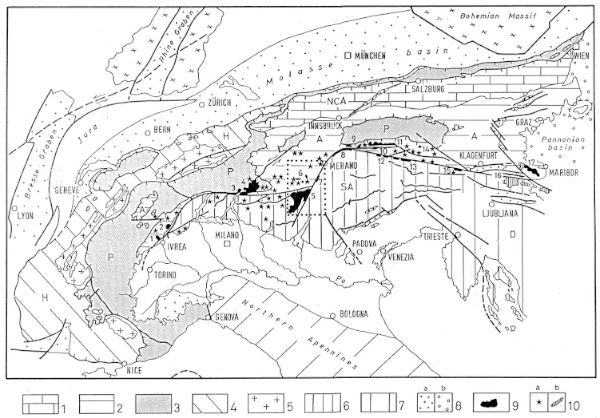
Tectonic map of the Alps and location of Tertiary magmatic bodies along the Periadriatic belt (Dal Piaz et al., 1988): 1) Austroalpine decollement nappes of Northern Calcareous Alps (NCA); 2) Austroalpine basement and cover units (A); 3) Penninic nappe system (P), icluding undifferentiated flysch and ophiolite units; 4) Helvetic-Dauphinois decollement nappes and sedimentary cover (H); 5) Helvetic-Dauphinois basment units; 6) Southern Alps (SA); 7) Dinarides (D); 8) Oligocene and Neogene sedimentary basins: 8a) Bresse-Rhine graben system, Molasse foredeep, Piedmont-Langhe and Gonfolite basins, eastern Alpine intramontane basins; 8b) Vienna, Graz and Pannonian basins. Periadriatic Periadriatic magmatism: 9) intrusive bodies; 10a) dykes; 10b) flows, tuffs and volcaniclastic cover. Main plutons: 1. Traversella, 2. Biella, 3. Novate, 4. Bergell, 5. Adamello, 6. Gran Zebrù, Mare valley and Lago Verde, 7. Rumo and Samoclevo lamellae, 8. Bressanone border lamella, 9. Rensen and Mt Alto, 10. Cima di Vila, 11. Vedrette di Ries, 12-15. Lamellae and apophyses along eastern Periadriatic line, 16. Karawanken, 17. Pohorje.
New geochemical and isotope data on some post-metamorphic lamprophyres cutting the Sesia-Lanzo zone (Owen, 2007) confirm their derivation from a metasomatized upper mantle source, as previously pointed out by Dal Piaz et al. (1977) and Venturelli et al. (1974). A questionable Rb-Sr isochron age of 44.2 ± 2.4 Ma has been obtained by Babist et al. (2006) from a trachyandesitic dyke which cuts the composite regional schistosity (S1+S2) and shear zones of the Sesia-Lanzo Eclogitic complex, ten years older than the well defined age of the post-metamorphic magmatism throughout the internal Western Alps, including the nearby Ayas valley.
Deep structure of the Eastern Alps
The detached Piedmont (European) slab beneath the Alpine retro-wedge, early suggested by the CROP-ECORS profile (Nicolas et al., 1990; Polino et al., 1990), was imaged by tomographic sounding (Lippitsch et al., 2003; Kissling et al., 2006) and corroborated by petrologic estimates suggesting a decrease of the thermal gradient between 50 and 44 Ma, probably due to a consistent increase of sinking velocity (Li et al., 2004; Groppo et al., 2009).
Passing to the Eastern Alps, a decisive step in reconstructing the deep structure of the collisional belt was given by the Transalp experiment, made by the joint efforts of Austrian, German and Italian working groups, the logical and long-awaited conclusion of CROP and NFP-20 deep seismic sounding across the Alps. This project carried out between Munich and Venice (1998-2001) simultaneous vibroseismic and explosive source recording, integrated by passive cross-lines (Bleibinhaus & Gebrande, 2005; Lüschen et al., 2006). The Penninic nappe stack exposed in the Tauern window is imaged as a huge, south-vergent antiform above the Late Oligocene-Neogene tectonic ramp separating the former collisional wedge from the subducting continental crust of the European foreland down to about 55 km below the Dolomite area. The inner collisional zone and the Southalpine domain are characterized by thick-skinned back-thrusting at the crustal scale. Two interpretations of the seismic image have been envisaged by the Transalp Working Group (2002), showing that the structural features of the collided European and Adriatic continental crust could be consistent to wedging of the former into the latter (crocodile model, Lammerer et al., 2002) or viceversa (extrusion model: Castellarin et al., 2002, 2006) (Fig. 21). The extrusion model is favoured, since indentation of the rheologically soft Penninic wedge into the relatively colder and more rigid Austro-Southalpine crust can be hardly sustained. It is also supported by the negligible Neogene uprising of the Dolomites, 6−7 times smaller than that estimated in the Tauern window. Therefore, the tip of the Southalpine lower crust is thought to have been moved against the ductile Penninic-Austroalpine wedge, along the northward dipping Periadriatic line, imaged to a depth of at least 20 km (Castellarin et al., 2006).
Figure 21. Transalp deep seismic profile.
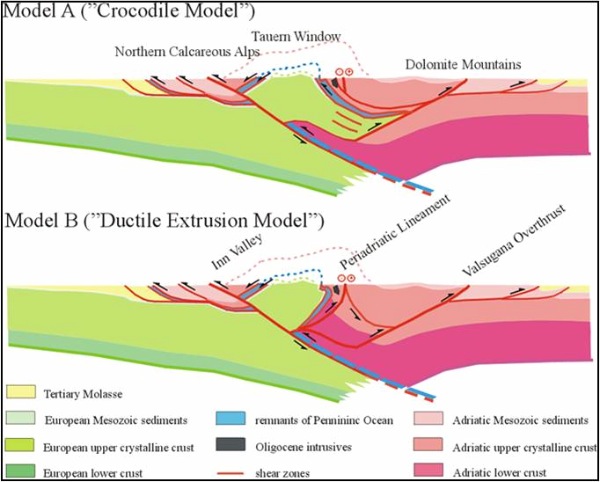
Contrasting interpretations of the Transalp deep seismic profile across the Eastern Alps: A) Crocodile model based on Pennidic wedging into the Adriatic lithosphere (Lammerer et al, 2002); B) Extrusion Model based on Adriatic indentation or northward protrusion (Castellarin et al., 2002).
The combination of nonlinear inversion, high-quality teleseismic data, and a 3D crustal model allowed a reliable resolution of cells at 50 km × 50 km × 30 km (Lippisch et al., 2003), hence structures as small as two cells can be resolved in the upper mantle. The tomographic images elaborated in this way by Lippisch et al. (2003) penetrate the upper mantle to 400 km depth, showing a high-velocity body that dips to the south-east beneath the Southern Alps in the Western and Central Alps. Anyway, the southward subduction of the European continental and oceanic lower plate along the entire Alps has been generally confirmed (e.g., Bleibinhaus & Gebrande, 2005; Castellarin et al., 2006; Lüschen et al., 2006).
Pre-Alpine evolution of the Grand St Bernard (Briançonnais) nappe system
The recurrent debate on the monocyclic (post-Variscan) vs polycyclic units, i.e. the “younger” and “older” basement in the Grand St Bernard (Briançonnais) nappe system, was enlivened by new dating of protoliths from various tectono-metamorphic units. Disregarding the Alpine blueschist and/or greenschist facies overprint, I refer to igneous bodies generally regarded as post-Variscan or Variscan in classic model of the French-Italian Alps (e.g. Ellenberger, 1958; Elter, 1960; Bearth, 1982; Platta & Lister, 1985; Escher, 1988; Desmons & Mercier, 1993), becoming older as follows: 1) Early Carbonifeous intrusion age of the Grand Nomenon metatonalite (356 ± 3 Ma; Bertrand et al., 2000a); 2) Middle Cambrian emplacement age of metagranophyres from Mont Pourri (507 ± 9 Ma; Guillot et al., 1991) and Rhemes valley (511 Ma ± 9 Ma; Bertrand, 2000a-b); 3) Cambrian-Ordovician U-Pb zircon ages (Bertrand et al., 2000b) of the Peclet orthogneiss (482 ± 5 Ma), Modane-Sapey metagranite (452 ± 5 Ma) and Ambin metarhyolite (500 ± 8 Ma), showing that a major igneous and tectonic cycle developed at 450-500 Ma, with little evidence for a Variscan imprint well documented in the near Helvetic basement (Von Raumer & Neubauer, 1993; Frey et al., 1999). Similar results and conclusions have been achieved in the Swiss side of the Grand St Bernard nappe system. After the new definition of the Siviez-Mischabel and Mont Fort nappes (Escher, 1988), the complex tectono-stratigraphic reconstruction was made more problematic due to the discovery of first-rank shear zones across the nappe stack and isotope dating of mafic and felsic igneous protoliths (504 ± 2 and 500 Ma ± 3: Bussy, in Sartori et al., 2006). Below the Meso-Cenozoic Briançonnais cover, seven metamorphic units can be recognized (Sartori et al., 2008), ranging from the Bruneggjoch (Eotriassic-Late Permian) and Col de Chassoure (Permian) monometamorphic formations to the Ergischhorn complex and Adlerflüe formation (probably Protérozoic), through the Lirec, Distulberg and Métailler formations (Cambrian ± Ordovician). Inherited zircon cores yielding 476 Ma and older ages have been recently dated within the calc-alkaline volcanic units of Permian (285-258 Ma) age occurring in the southernmost Briançonnais domain of the Ligurian Alps (Dallagiovanna et al., 2009). All this opens the intriguing question on the possibility that the Brianconnais basement units underwent Cambrian events (magmatic and tectono-metamorphic) and escaped the Variscan orogeny, similar to the far French Massif Central and opposed to the near Helvetic-Dauphinois domain (e.g., Malusà et al., 2005).
Western Austroalpine and ocean-continent transition
Back to the Western Alps, some contributions are really innovative, mainly through refinement of petrological estimates and new isotope dating on retentive systems, confirming the age of subduction metamorphism in the Western Austroalpine (Late Cretaceous) and in the Penninic nappe systems (Eocene), oceanic units included, as well as on their paleogeographic reconstruction. I refer to papers by Beltrando et al. (2010b) and Angiboust & Agard (2010) on the lower Aosta valley, and to the monography by Beltrando, Compagnoni & Lombardo (2010a) on the high-P metamorphism and the orogenic evolution of the entire Western Alps, that I have seen when long I was struggling with this Alpine review.
Roisan zone - Focusing on the Roisan zone, generally referred to as a detached metasedimentary cover unit of the upper Austroalpine Dent Blanche-M.Mary thrust system (Hagen, 1948; Diehl et al., 1952; Elter, 1960; Compagnoni et al., 1977; Ayrton et al., 1982; Escher et al., 1997; Dal Piaz, 1999; Steck et al., 1999, 2001; Bucher et al., 2003-04), it can be mentioned the discovery of a rich association of Late Triassic benthic foraminifers, occasionally preserved within dolomitic bodies in the Grand Pays range, high St Barthélemy valley (Ciarapica et al., 2010). A comparative work (Passeri et al., in progress) shows the important lithostratigraphic differences between the Roisan and Mt Dolin successions (Fig. 22).
A pending problem is the unique outcrop of banded micro-quartzites discovered by Ballevre & Kienast (1987) inside the Arolla series, over the Cignana lake, referred to the Mesozoic metasedimentary cover of the Dent Blanche nappe (Roisan Zone), and interpreted as a metamorphic derivative of a Mn-rich and oxidized siliceous deposit (metaradiolarite?). This is a metric bed included within a folded and boudinaged horizon of calcschists and impure marbles, often mylonitic, consisting of the alternance of white, black, pink and purplish thin quartzitic layers with various amounts of almandine and spessartine-rich garnet, hematite, blue amphiboles (crossite-magnesioriebeckite), Fe-epidote, Piedmontite, chlorite, biotite, stilpnomelane, apatite, allanite, titanite. Preliminar SHRIMP dating on allanite (Manzotti et al., 2009) provided Permian (ca 280 Ma) and scattered Jurassic ages (190-160 Ma), whereas titanite in associated marbles yielded apparent spot ages between 284 and 160 Ma. The hydrothermal mineralization and the Jurassic age point for an oceanic environment, and thus for a Piedmont affinity because i) similar Mn- and Fe-rich quarzites are relatively abundant within the underlying Combin Zone (Dal Piaz & Omenetto, 1978; Dal Piaz et al., 1978), ii) the post-Triassic Dolin-Roisan sequences are represented by micritic marbles and polymict sedimentary breccias (Hagen, 1948; Ayrton et al., 1982), iii) no further Fe-Mn quartzites have been recognized throughout the Roisan zone, recently mapped at 1:10.000 scale (Foglio Monte Cervino of the 1:50.000 Carta Geologica d’Italia, data set at Regione Autonoma Valle d’Aosta). The allanite Permian ages (Manzotti et al., 2009), if confirmed and geologically meaningful, could be referred to clastic materials supplied by eroded granitoids of the Arolla series that yield a similar primary age (Bussi et al. 1998), making questionable, in this case, the favoured Piedmont affiliation.
Figure 22. Roisan and Mt Dolin metasedimentary cover units.
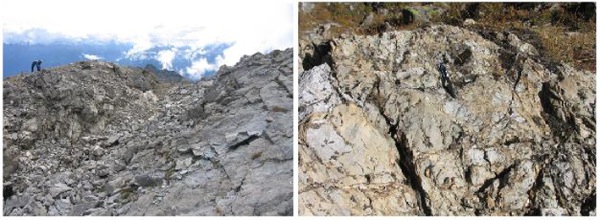
Left – Collapsed Grand Pays summit (trench), consisting of dolomitic marble and breccia with Late Triassic foraminifers (Ciarapica et al., 2010). Right - Mt Dolin: sedimentary breccia of assumed Jurassic age (Hagen, 1948; Ayrton et al., 1982).
Permian gabbros - The already envisaged Permian age of the monometamorphic Matterhorn-Mt Collon gabbros (Fig. 23) inside the Arolla series of the Dent Blanche nappe (Dal Piaz et al., 1977) has been confirmed and refined by Monjoie et al. (2007), providing concordant U-Pb zircon ages of 284.2 ± 0.6 Ma and 282.9 ± 0.6 Ma for the layered mafic complex of the Mt Collon body and a crosscutting leucocratic dyke, respectively, togheter with a 40Ar/39Ar age of 260.2 ± 0.7 for a later Fe-Ti-rich lamprophyre. Similar Early Permian ages had been obtained by Bussy et al. (1998) for the protoliths of the Sermenza metagabbro (288 Ma), north-eastern edge of the Sesia-Lanzo zone, and the gneissic granitois of the Italian southern face of the Matterhorn (289 ± 2 Ma).
Sesia-Lanzo zone - Working on the entire Sesia-Lanzo Zone, Babist et al. (2006) corroborated the concept that this composite nappe system is a relic of the subducted part of the Adriatic continental margin along the internal boundary of Tethyan ocean (Dal Piaz et al., 1972; Compagnoni et al., 1977). Three basement nappes have been recognized therein, that developed during the Late Cretaceous (80-65 Ma) subduction-related reworking of shear zones, early generated by continental rifting, i.e.: i) Bard nappe, mostly corresponding to the Gneiss minuti complex (Gastaldi, 1871-74), ii) Mombarone nappe, corrsponding th the Eclogitic micaschists complex (Stella, 1894), iii) IIDK, high-grade basement rocks similar to the diorito-kinzigitic Ivrea Zone (Artini & Melzi, 1900). The Bard and Mombarone nappes are partly separated by a discontinuous horizon of Mesozoic metasedimente (Bonze unit; Venturini, 1995). Five Alpine deformations phases and six fabric domains have been distinguished and schematically figured. New Rb-Sr mineral dates constrain the age of D2 transpressional blueschist shearing D2 (60–65 Ma) and D3 extensional greenschist facies shearing (45–55 Ma), indicating that, in Eocene times, top-SE extensional exhumation of Sesia-Lanzo was coeval with SE-directed subduction of the Ligurian-Piedmont oceanic lithosphere. This reconstruction goes against the recurrent hypothesis of exhumation through an extensional phase developed after any high-P metamorphic event in the Austroalpine-Penninic collisional wedge (e.g., Ballèvre & Merle, 1993; Avigad et al., 2003; Rosembaum & Lister, 2005). In particular, the latter authors envisaged a widespread phase of tectonic extension troughout the collisional belt at about 42–38 Ma, thought to be induced by a rapid retreat of the subduction hinge below the accreting Grand St Bernard (Briançonnais) nappe system (Stampfli et al., 1998; Stampfli & Hochard, 2009).
Figure 23. Geology of Italian southern face of the Matterhorn.
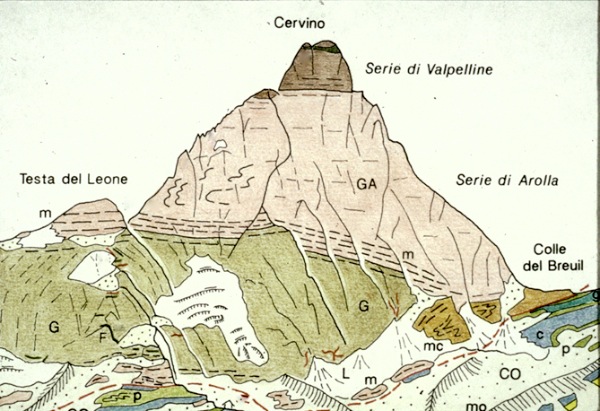
From top to bottom: mainly retrogressed kinzigitic complex of Valpelline series; coarse- to fine-grained and folded gneissic granitoids of Arolla series (GA), from Permian protoliths; Permian gabbro (G) with a thick mylonitic horizon (m) between them and at the base; basal slice of micaschists; calcschists (c), prasinites (p) and mylonitic gabbros (dark green) in the underlying Combin zone (Dal Piaz, 1992; Bucher et al., 2003, 2004).
Ocean-continent transition in the lower Aosta valley – Three classic sections of the eclogitic Zermatt-Saas ophiolite (Mt Avic, Breuil-Ayas and Täsch, near Zermatt) have been carefully mapped and investigated by Angiboust & Agard (2010) for evaluating the extent to which the hydration of the oceanic lithosphere and related density decrease would have prevented the definitive disappearance into the subduction zone of detached high-P fragments of the oceanic lithosphere, and assisted their rapid uplift along a serpentinized subduction channel. Large amounts of fluids were provided by sea-floor alteration and hydrothermal processes to oceanic basalts and mantle peridotites, now represented by various high-P mafic rocks (lawsonite eclogites, glaucophanites, garnet-chloriteschists) and serpentinites. Additional references may be suggested for the Italian areas, concerning: i) Savoney-Mezove (Avic) ophiolitic complex, at the base of the Glacier-Rafray continental basement slices: this zone, also mapped by Dal Piaz, Nervo & Polino (1979), was described in detail by Dal Piaz & Nervo (1971), including four whole-rock analyses of the same ophiolitic suite studied by Angiboust and Agard; ii) Breuil-upper Courtod valley (Ayas): see “From the European continental margin to the Mesozoic Tethyan ocean: A geological map of the upper Ayas valley (Western Alps)” (Dal Piaz, 2004), in “Mapping Geology in Italy”, by APAT-Servizio Geologico d’Italia.
The eclogitic lower Austroalpine Etirol-Levaz slice has been studied and dated by Beltrando et al. (2009b, 2010b). A mylonitic orthogneiss from the bottom of the slice, near the contact with the underlying serpentinites and gabbros of the Zermatt-Saas zone, and an eclogitic boudin inside paraschists from the middle of the slice were analyzed, both providing in situ U-Pb zircon ages. The former sample yielded Permian and Jurassic (166–150 Ma) zircons, and the latter ages are attributed to melt infiltration associated with the intrusion of the underlying gabbros. Zircons from the eclogitic boudin yielded Late Permian ages (ca. 263-253 Ma) in zoned cores, and Eocene ages (47.5 ± 1.0 Ma) in unzoned rims, like in several previously quoted eclogitic occurrences of the Zermatt-Saas zone. In spite of the existence of a tectonic contact between the continental and oceanic units, the interpretative model proposed by Beltrando et al. (2010b) suggest the existence of a primary lithostratigraphic association of mantle serpentinites, continent-derived allochthons, Jurassic oceanic gabbros and post-rift sediments, typical of an ocean-continent transition, and probably representative of a hyper-extended margin related to Tethys opening. This heterogeneous crustal association, acquired before the Alpine orogeny, was characterized by a negative buoyancy that facilitated, as pointed aut in previous works (e.g., Dal Piaz, 1999), the onset of lithospheric subduction.
Subduction metamorphism and orogenesis: a last Alpine perspective.
The monography by Beltrando, Compagnoni & Lombardo (2010) on the high-P metamorphism and the orogenic evolution of the Western Alps is a detailed, updated and well documented contribution that I am pleased to agree with as a whole (not only for the kindness with which they have reported my previous work). The Western Alps are described as the amalgamation of a Cretaceous and an Eocene orogen, which developed at the expense of the Adriatic and European rifted margins. This concept is supported (apparently) by the gap of about 30 Ma between the subduction age of the Sesia-Lanzo and upper Austroalpine outliers and that of the Zermatt-Saas, Monte Rosa and Gran Paradiso nappes. I am sure, however, that authors share the paradigm that, instead of two distinct orogens, in the Western Alps there is only a lack of surface records (lithotectonic bodies and/or isotope dating and partitioning effects), or of information on lesser appealing non-eclogitic units, since plate convergence was a continuous global process at least from the “mid” Cretaceous. It is right that the Combin and Zermatt-Saas ophiolitic units were juxtaposed during exhumation in the Late Eocene (38 Ma), but is not documented and unlikely that these oceanic fragments underwent their contrasting pressure peaks at the same time (40-44 Ma): it can be recalled that the age of the poorly preserved blueschist facies relics in the Combin zone across the Aosta valley and southern Valais is essentially unknown, but it is probably older (at least in places) than the eclogitic imprint in the Zermatt-Saas nappe, since the former acted as tectonic sole of the upper Austroalpine units during their accretion-exhumation history. Moreover, it can be remembered the evidence of orogenic deposits accumulated in the outward migrating oceanic trench and later displaced in various decollement nappes (see Caron et al., 1989, and Polino et al. 1990, for reviews). Hence, in my opinion the paradigm that the Alpine orogeny is a discrete process related to the episodic accretion and exhumation of continental and oceanic units (e.g., Rubatto et al., 1998; Gebauer, 1999; Rosenbaum & Lister, 2005) is a reductive and unilateral view. I thank very much the authors (and also Handy et al., 2010) for having attributed the original idea of the so-called “tectonic erosion” to the subduction model elaborated by Dal Piaz et al. (1972), but I do not believe that this is actually true, at least in the sense of Polino et al. (1990), a theory not yet conceived at that time and in any case unkown to me. In subsequent papers (Dal Piaz, 1974; Compagnoni et al., 1977; Dal Piaz & Polino, 1989), the boundary of the convergent plate margin at the incoming subduction is mapped along the contact between the Austroalpine and Southalpine domains, becoming intraoceanic within the Ligurian basin, allowing the obduction of the Ligurian ophiolitic nappe. 4) Among the main four age groups of high-P minerals recognized in the Western Alps (70Ma; 55 Ma; 48–42Ma; 38–33 Ma) after more than four decades of extensive geochronological studies, the second one (ca 55-47 Ma) deserves a short comment. It is based on high-P zircon and allanite dating from the Lanzo massif (Rubatto et al., 2008) and K-Ar white mica ages from the detached Mesozoic cover (Mt Dolin) of the Dent Blanche nappe (Ayrton et al., 1982), with a large spread from 55 to 34 Ma. Whilst the former is a robust dating of the high-P metamorphism in a slice of oceanic lithosphere, the latter probably groupes mixed to greenschist facies ages as it can be inferred from the Rb-Sr white mica mixed (57 ± 6, 52 ± 1.3) and blueschist facies (75-74 Ma) ages recorded at the same strutural level in the frontal part of the Sesia-Lanzo Gneiss minuti complex and in the Pillonet klippe, respectively (Cortiana et al., 1998). I shall also mention that the Rb-Sr and 40Ar-39Ar dates of Cortiana et al. (1998) on the Sesia-Lanzo mylonitic metagranitoids in the Ayas valley are ignored by Babist et al. (2006).
The new Geological Map of Italy
Our historical journey is ended and it may be concluded with some informations and comments on the new Carta Geologica d’Italia at 1:50,000 scale (Progetto CARG). The project started in the 1988 and it was based on the cooperation between the Italian State (SGN-ISPRA) and the Regions, involving more than 60 local authorities, CNR Institutes and University Departments. The mapping project consists of 652 geological sheets, surveyed at 1:10,000 scale and completed with sections, explanatory notes and digital database. Only 257 sheets are currently printed or in progress, covering 40% of Italy (for the state of the art, see the site ISPRA, Progetto CARG). In the Western Alps only the Bardonecchia, Chatillon, Torino, Sanremo and Susa sheets are printed, and in the Eastern Alps the sheets Adamello, Ampezzo, Appiano, Asiago, Cortina, Malè, Maniago, Merano, Rabbi, Riva del Garda, San Vito al Tagliamento. Tione, Trento, Udine. The great delay has many causes and is often attributable to the authors, but also to national and local authorities. For instance, the Vetta d’Italia sheet was printed as a 1:25,000 regional map in the 2004, but the 1:50,000 sheet (003) and its explanatory note, long ready for printing, have not been appeared yet only for an inexplicable negligence of the Provincia Autonoma di Bolzano. Anyway, even sadder for the degraded national environment is that most of the maps have not been assigned yet. The completion of the mapping project is a primary need, since a modern geological sheet and its database are the unavoidable groundwork for a correct recognition of natural risks and their mitigation.