In most of the Central-Iberian autochthon the main Variscan shortening induces a pervasive folding that is usually the most notorious structural feature. The geometrical behavior of these D1 folds, well exposed in the Marão sector (Fig. 6), strongly depends of the deformed lithostratigraphic units.
Figure 6. Geological map
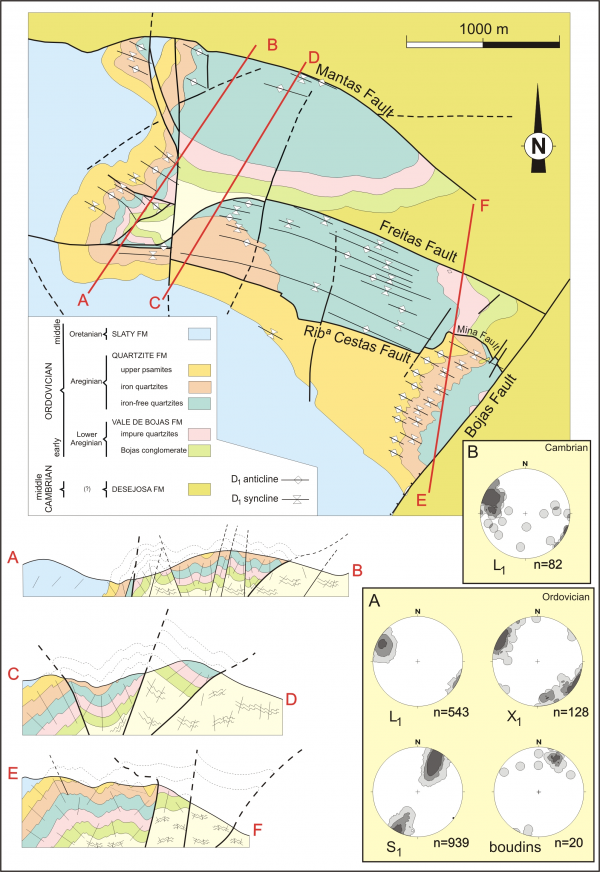
Geological map of Marão studied sector, interpretative cross sections, and contoured equal area lower hemisphere stereographic projection for main D1 structures.
The folds developed in post-Cambrian metassediments present a very constant geometry (Fig. 6A):
WNW-ESE axial plane dipping 70º to 80º to southwest showing a northeast facing;
fold axes plunging less than 10º to WNW;
intersection lineations (L1) between the coeval cleavage (S1) and the bedding plane always subparallel to the fold axes, emphasizing an axial plane cleavage;
a subhorizontal WNW-ESE stretching lineation (X1).
The very low angle between the fold axis and the stretching lineation denotes a stretching subparallel to the kinematic b axis, a common feature in most of the Central Iberian autochthon in northern Portugal (Ribeiro, 1974; Ribeiro et al., 1990; Dias, 1994; Coke, 2000). In the Marão sector the necking of most of the boudins tends to be subperpendicular to the fold axes (Fig. 6A) which is compatible with the previous described kinematics.
However, when the folds are in the middle Cambrian metasediments of the Desejosa Formation their geometry is more variable. Although the outcropping conditions are now poor, the wavy pattern of the D1 folds is clearly expressed by the more dispersed behavior of the L1 Variscan lineation (Fig. 6B).
The above fold geometries can only be explained assuming a pre-ordovician deformation. This event, known as Sardic phase, has been a weakly one as could be deduced by the open fold geometries and the absence of a coeval cleavage (Ribeiro, 1974; Ribeiro et al., 1990). Such a behavior could be explained by a transient inversion during the rifting stages of the Variscan cycle (Romão et al., 2005).
Major discrete shear zones having a low angle to the axial planes are frequent (e.g. Mantas, Freitas and Ribeira das Cestas faults). These cartographic structures are clearly contemporaneous of the Variscan D1 folds, as could be deduced by the increasing of the S1 cleavage in the vicinity of the shear zones. Concerning their kinematics, they are essentially major sinistral wrench faults (Dias and Ribeiro, 1994; Coke et al., 2003), as shown by different geological markers: e.g. C/S minor structures, en echelon minor folds, sigmoidal distortion of the conglomeratic pebbles, deflection of the Skolithos worm burrows and oblique slickensides along active bedding planes (Fig. 7).
Figure 7. Shear Zone
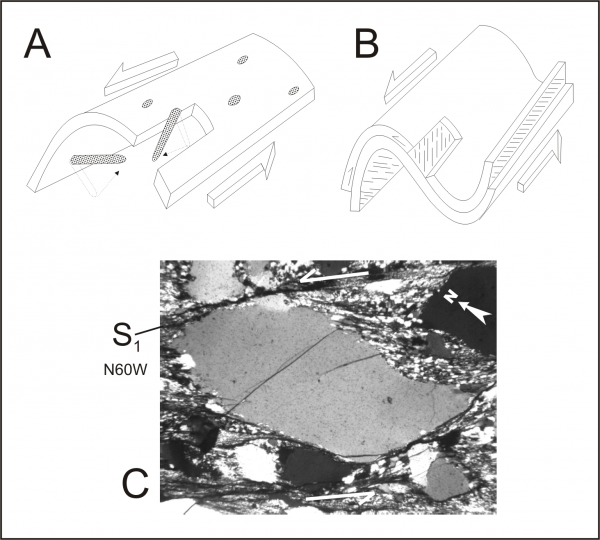
Regional sinistral shear component expressed by the deflection of Skolithos and oblique striae on bedding plane.
The previous structural pattern shows that the sinistral oblique collision dominant in the south branch of the Ibero-Armorican Arc (Dias and Ribeiro, 1994, 1995; Ribeiro et al., 1995) have been partitioned: the shortening component were concentrated in the folds, while most of the regional sinistral component is found in the vicinity of the major shear zones.
To compare with the former described structural style in Lower Parautochtonous Domain, the geometry and kinematics of D1 structures in Upper Parautocthonous is done considering an area northeast Murça in the Serra de Santa Comba (Figs. 5 and 8).
References to the geometry and kinematics of the Variscan deformation in the parautochthon of NE Portugal are rare (e. g. Ribeiro and Rebelo, 1966; Ribeiro, 1970, 1974; Ribeiro et al., 1990). However, the Murça sector is a privileged one for deciphering the D1 macrostructure due to the large cropping areas of silurian quartzites. These rocks have the ability to preserve primary structures (Fig. 9A) allowing the easy definition of the D1 facing based on the fold style and orientation. The high ductility contrast between the quartzitic layers and the adjacent ones of phyllitic nature concentrates the post-D1 ductile deformation in the phyllites preserving the D1 structures in the quartzites.
Figure 8. Geological map
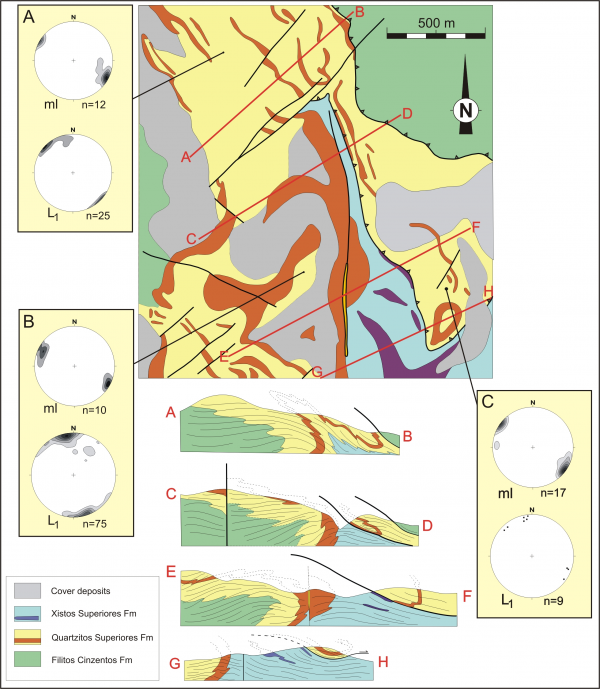
Geological map of Upper Parautochthonous studied sector, interpretative cross sections, and contoured equal area lower hemisphere stereographic projection for D1 intersection lineation (L1) and mineral lineation in S1 (ml).
Mesoscopic D1 folds are very scarce and only with detailed mapping are possible to define their geometry. These folds, always verging to E or SE, are recumbent with very tight to isoclinal style and sub-horizontal axial planes. Some “tête plongeante” cases are defined. Associated with the D1 folding phase a pervasive S1 slaty cleavage occurs (Fig. 9B). This cleavage is parallel to the axial plane of the D1 folds, and no signs of transection were found. The hinge orientation is thus determined by the intersection lineation, and exhibits an arcuate pattern even at cartographic scale; the studied sector is located in the northern part of one of this major arcuate folds. Although not always present, a mineral lineation is common in S1; this lineation plunges less than 20º to ESE direction (Fig. 8A to C).
Figure 9. Field photos
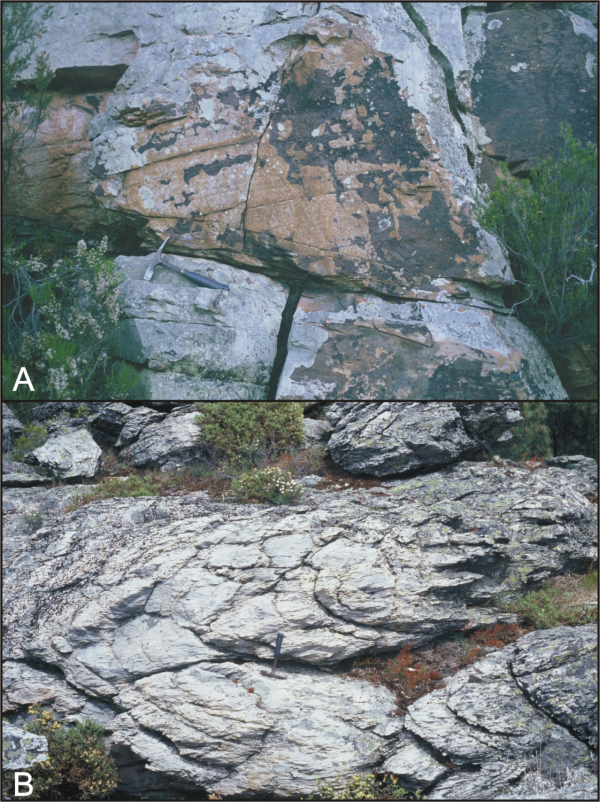
Some details of the parautochthonous Silurian quartzitic rocks: A- primary cross-bedding; B- sub-horizontal axial plane S1 slaty cleavage.
The meaning of this mineral lineation should be considered together with the spatially related mylonitic foliation, which predominates in the reverse limbs of the D1 folds. Both structures are parallel to the S1 foliation and associated mineral lineation. In Figure 4 geological cross sections, the thrust planes parallel to the D1 axial planes have been possible to map due to the presence of this mylonitic foliation (Fig. 10). The mylonitic strips associated with the D1 reverse limb are rooted northwards in an undisrupted D1 macrofold. These emphasize a genetic association of mylonitization and D1 folding phase in a highly heterogeneous strain regime.
Figure 10. Photomicrograph
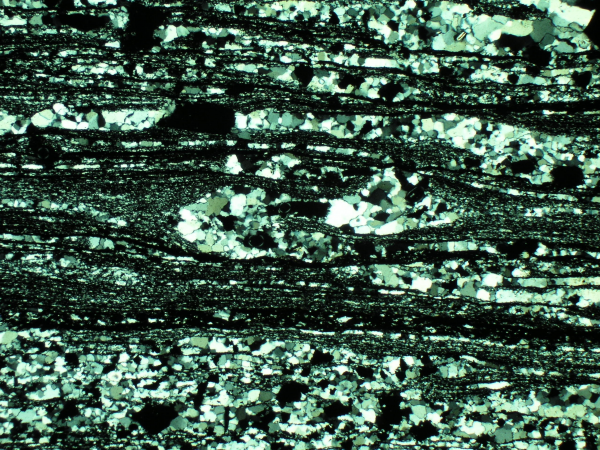
Photomicrograph of the D1 mylonitic foliation showing rootless intrafolial folds. Black cherts level of Xistos Superiores Fm represented in cross-sections E-F and G-H immediately under the thrust plane (crossed polars, base of photo 4 mm).
This geometry, as well as the existence of basal Palheiros - Vila Flor Trust plane with several tens of kilometers of displacement, suggest the presence of a structural style characterized by large fold nappes, with alpine helvetic style, but unrooted. In the studied sector, these folds have a SE facing, which is parallel to the transport deduced from the mylonitic lineation and associated kinematic indicators that indicate a top to SE nappe emplacement.
Concerning the geometry of the D1 structures some features should be emphasize (Fig. 11):
they tend to occur as rootless fold-nappes with axial planes parallel to nappe basal thrust and curved hinges with an arcuate pattern at map scale;
the deformation regime is far from plane strain deformation
the heterogeneous nucleation of shear zones rooted in the reverse limbs of D1 macrofolds is common.
In these macrofolds a strong strain heterogeneity is evident. It is possible to observe from cross bedding in the quartzitic levels (Fig. 9A) to mylonites of black cherts immediately below (Fig. 10). In the quartzitic levels, the fold type and the coeval slaty cleavage points out to a strain regime dominated by the pure shear component normal to the nappe basal thrust plane. However, in the reverse limbs of the macrofolds, this situation turns into a strain regime dominated by simple shear just below the quartzitic layers, especially in the black shales and cherts. These facts indicate a strain partitioning controlled, not only by the strain variation in the fold, but also by the rheology of the rocks.
Usually the simple shear component becomes more intense in the lithologic levels with higher content of phylossilicates. In the units without quartzites the bedding is always completely transposed.
The understanding of the processes that generate the fold axis curvature is also a crucial one. In the absence of finite strain data two main models could be used in order to discuss the relations between fold axis curvatures, maximum finite stretching and transport direction (Ribeiro, 1974):
the maximum finite stretching is parallel to the fold axis. In this case the material flow is divergent;
the maximum finite stretching shows a convergent pattern into the zone of the maximum curvature of the fold axis arc. In this case variable angular relationships with the fold axis are possible.
Where the structure is dominated by the pure shear component, namely in the massive quartzitic levels, the observation of thin sections parallel to the regional S1 cleavage, as well as the elongation in conglomerate clasts, show a preferential elongation of the original sand grains and pebbles parallel to the local intersection lineation. This strain pattern reflects a typical divergent material flow without lateral constriction. In these levels, the mineral lineation is absent.
With the increasing content of phylossilicates, the slaty cleavage occurs, became more and more evident being conspicuous in the phyllitic units. In those cases a mineral lineation is common, being parallel to the lineation found in the mylonitic foliation associated with the D1 reverse limbs. The main rheological consequence of this lithological change is the onset of the simple shear component that culminates with the mylonitic foliation of the reverse limbs of the D1 macrofolds.
Thus, in the more quartzitic levels with low mica content, the plane of maximum finite shortening is materialized by the S1 slaty cleavage, and the maximum finite stretching is parallel or subparallel to the fold axis.
In the levels where the simple shear predominates, the mineral lineation and the mylonitic lineation represent the maximum finite stretching and also the transport direction of the nappes, with a top to SE movement in the studied sector.
The pattern of the stretching direction in the sectors of the nappe pile where the pure shear component predominates is compatible with a spreading dominant model of nappe development (e.g. Merle, 1994; Ramsay and Lisle, 2000). This spreading without lateral constriction develops together with gliding mechanisms, concentrated and controlled by particular lithologic levels, such as the black shales and cherts.
In this interpretation, the first order macrofold, represented by the Garraia – Santa Comba Arc, with its arcuate pattern is a primary spreading structure, despite the possibility of its enhancement during D2 Variscan events.
To conclude this kinematic approach to the D1 deformation in parautochthon, it should be emphasize that factors like the strong ductility contrast between different lithologies and strain variation inside D1 macrofolds explain the highly heterogeneous distribution pattern of the mesoscopic structures, such as cleavage types and intersection lineation curvature. These factors account also for the explanation of strain partitioning between a dominated pure shear component normal to nappe basal thrust plane and a simple shear component parallel to it.