Models to explain the evolution of the Eifel-Ardennes mullion structures fall into a number of classes summarised below. This is not a complete list, but a summary of the main classes.
Model (1): The structures formed by layer-parallel extension only (Corin, 1933; Wegmann, 1932).
This model fails to explain many of the observations, for example the high angle between bedding and cleavage, and was rejected long ago.
Model (2): The intra-mullion veins are formed late, after the formation of the cuspate-lobate structures (Quirke, 1923)
This calls for spontaneous nucleation of the cuspate-lobate structures at the layer interface (Ramsay and Huber, 1987; Ramberg, 1979) or at load cast structures (Jongmans and Cosgrove, 1994). This phase is followed by further shortening and formation of cleavage. At a later stage, formation of veins is initiated at the cusps, perhaps during a stress relaxation event (Mukhopadhyay, 1972). This model does not explain the deformation features in the veins (pinch-and-swell structures, undulose extinction) (Sintubin et al., 2000), the layer thickness dependence of the size of the mullions (cf. Tromme, 1997), and the shape of the mullions with cuspate-lobate folds symmetric on both sides of the layer. Also, it is not clear why in this model the veins should stop at the psammite-pelite interface (the cleavage has already developed in the pelite). In addition, the nonzero angle between mullion axes and the delta lineation is a strong argument against this model.
Figure 14. Structural data
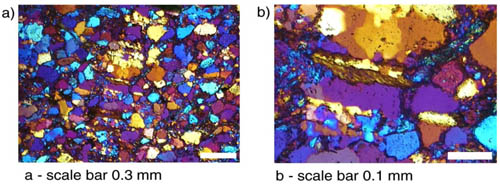
Structural data (lower hemisphere, equal area) from selected groups of outcrops from the study area. (a) Outcrops with delta lineation close to the mullion axis. (b) Example of a case where these two lineations are clearly distinct. Lower hemisphere, equal area projections. Legend: open circles- Mullion axis; open triangles- poles to intramullion veins; open squares- poles to bedding; crosses- poles to cleavage in pelite; dots- delta lineation (intersection of bedding and cleavage). (Click for enlargement)
Model (3): The intra-mullion veins formed during the early stages of layer-parallel shortening, before the main development of folds and cleavage (Bruhl, 1969; Sintubin et al., 2000).
In the model of Brühl, the growth of crystals in the veins is thought to occur due to the force of crystallization. Although this is an exciting idea which recently has been further developed (Wiltschko and Morse, 2001), in our case it seems unlikely because the orientation of intra-boudin veins implies opening of the vein against the largest principal stress, and parallel to the largest tensile strength. It is much more likely that the force of crystallization would have opened the veins parallel to layering. In addition, the vein microstructure (syntaxial) does not agree with the force of crystallization arguments either. Final arguments against this model are the regularity of the structures and the nonzero angle between intra-mullion veins and cleavage.
Model (4a): The intra-mullion veins formed before the start of layer-parallel veins, as boudin-necks during an extension event (Jongmans and Cosgrove, 1994; Rondeel and Voermans, 1975; Tromme, 1997). The present shape of the structures is a deformed equivalent of the early boudins, but the cuspate-lobate shape is caused by the early boudinage. This was presumably the argument of Jongmans and Cosgrove (1994) for using the term boudin.
Figure 15. Stereogram
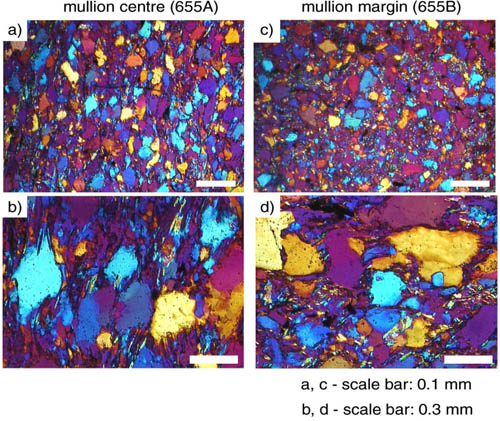
Stereogram showing all delta lineation and mullion axis data from the study area. Insert shows a cross-plot of the azimuth of these two datasets. Lower hemisphere, equal area projection. (Click for enlargement)
This model is consistent with the location of veins in the sandstone layers, the deformed veins, the cleavage patterns and the angle between veins and delta-lineation. It has problems, however, with the aspect ratio of the mullions, which would require unrealistically high shortening strains (Sintubin et al., 2000). In addition, the 3D shape of boudinaged layers is usually much more irregular than observed in this study, even considering the later deformation which tends to make structures more cylindrical.
Model (4b): The intra-mullion veins formed before the start of layer-parallel shortening, in a regionally developed joint system during events of hydraulic fracturing (Sintubin et al., 2000; Hilgers et al., 2000). During later shortening the veins were mechanically stronger than the sandstone, and the veins acted as stress guides for extrusion of the sand normal to the layers (Fig. 17). During later shortening, the folds and the cleavage were formed, and the mullions became asymmetric in the fold limbs.
The key elements of his model were developed simultaneously and independently in early 2001 by Kenis et al. (2001) and Urai et al. (2001), see also Kenis et al. (in press).
Figure 16. Histogram
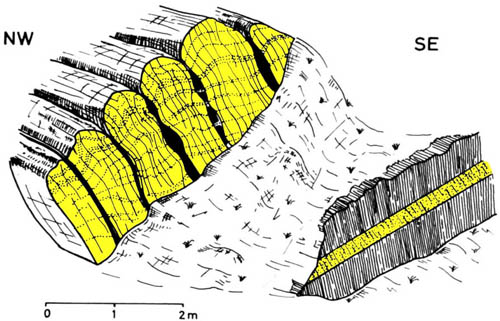
Histogram of the angle between delta lineation and mullion axis. (Click for enlargement)
This model does not disagree with the observations published so far, including our dataset. In what follows, we discuss some aspects of this model in some detail.
Regular, narrow-spaced fracture sets perpendicular to bedding in layered siliciclastic sequences are a common feature. They can be locally very regularly spaced, and evolve into vein sets in the presence of supersaturated fluids. Examples are given in Price and Cosgrove (1990). Their preferred location in psammite layers is consistent with stresses measured in sandstone-shale sequences in sedimentary basins during burial, which consistently indicate lower horizontal stresses in sand than in clay. This means that with progressively decreasing horizontal effective stress during periods of pore pressure increase, mode I fractures and subsequent veins first develop in the sand layers, only occasionally penetrating into the slate. The lateral teminations of the mullions, which can be occasionally observed, are similar to the vein terminations observed in field studies. A more detailed discussion of the stress path in this region is presented in (Hilgers et al., 2000).
Microstructures (stretched crystals, layer parallel fibres) in the sandstone indicate that the sand was lithified before the fractures developed in a high fluid pressure environment. This overpressure event (Sintubin et al., 2000; Hilgers et al., 2000) was extensive and long-lived and was accompanied by a slight (5-10%) horizontal extension as indicated by the total amount of vein quartz in the psammite layers.
Figure 17. Model for the development of mullion structures
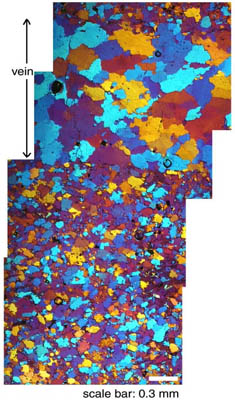
Model proposed for the development of mullion structures: (a) An illustration of the model proposed for the development of mullion structures.
The right hand diagram is a trace of the sample shown in Fig. 7e. The left-hand diagram shows an interpretation of the geometry of this structure at the beginning of layer parallel shortening. The image was constructed assuming constant volume deformation and no deformation of the quartz veins. As argued for in the text, at this point there was a clear competence contrast (vein quartz > psammite > pelite). The quartz veins thus reduced the vertical stretching in the psammite along their margins, causing the psammite to be extruded perpendicular to the layers. See text for discussion.
(b) Schematic illustration of the process by which mullions become asymmetric during progressive folding.
The mullions were then initiated during the early stages of sub-horizontal Variscan shortening. In this period, the strong veins acted as stress guides for extrusion of the sand normal to the layers. Arguments for the vein quartz being stronger than the sandstone are the fine grain-size of the sand and the evidence for solution transfer processes, and the CO2 and N2 - rich fluids in the quartz. It has been shown experimentally that milky vein quartz containing CO2 rich fluids is much stronger than milky vein quartz containing H2O-rich fluids (Dijkstra, 1990).
The angle between mullion axes and delta-lineations is a consequence of a coincidence between the early veins and the Variscan shortening direction. This was oriented close to but not exactly parallel to the opening directions of the early veins: In this orientation the mullions could easily form. According to the model mullions do not form when the shortening is at a small angle to the veins. Therefore a critical observation to falsify the model would be mullion axes at very high angle to the delta lineation. To date such outcrops have not yet been described.
Mullions thus formed early in the Variscan shortening, and became asymmetric during the later folding. In areas of the regional folds where bedding became sub-parallel to cleavage, the psammite layers which first developed the mullions presumably underwent a later phase of stretching, forming the boudins observed in these locations (Fig. 4c) (Spaeth, 1986).
The development in two different tectonic phases is consistent with the variable nonzero angle between mullion axis and the cleavage. During shortening the veins were effective in controlling the local strain field and perhaps had a small effect on the local orientation of the fold axes, but the direction of shortening and the development of regional fold sets and cleavage were controlled by regional forces.
Not all observations are equally well explained so far. For example, the spindle shape of the intra-mullion veins is not like the veins found in their undeformed equivalents. This is one of the questions which requires further study.