Experimental
Results
The
experimental models exhibited a wide spectrum of ramp/ flat thrust accommodation
styles mainly controlled by scale-effects and the rheological stratification,
as discussed below.
The scale effect of ramp-flat accommodation
In a first
model ( Fig.4a) a single plastilina block with induced ramp was detached
from a rigid base to simulate the scale effect and rheological control
of ramp-flat thrust accommodation. This was done simply by end-loading
models with similar initial set-up but under different g-values i.e. under
normal gravity and alternatively in a centrifuge (cf. Figs.4 b & c).
In the model end-loaded under normal gravity, because strength was not
properly scaled for gravity, the hangingwall block translated forward
without bending and unbending to the form of the footwall. As a result
void spaces developed during translation above fault bends.
By comparison
in the centrifuged model (Fig.4c), the special geometric features of ductile
fault bend folding developed from the necessity of making geometric adjustments
in the hanging wall to conform to the shape of the underlying flat-ramp-flat
footwall. This required a yield stress of the hanging wall blocks in near
balance with the gravity stress. In this particular model, because the
footwall was deformable a footwall synform developed in response to the
load imposed by the advancing hanging wall.
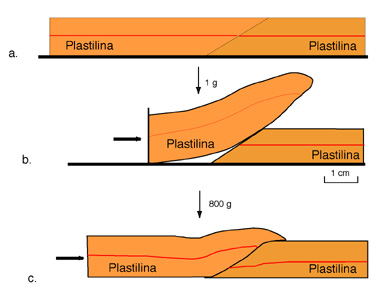 |
Figure 4.
a) Initial set-up of a single plastilina layer detached above a rigid
base. b) and c) represent deformed models under normal gravity and
in a centrifuge, respectively. (Select
image for enlargement) |
Ramp-flat
accommodation in a competent layer beneath ductile strata
Figures 5 and 6 show
various 2-layer models which show how the initial ramps changed shape
while slip accumulated along the flat and ramp sector, mainly controlled
by the rheological stratification. In a first arrangement (Fig. 5a) the
plastilina material, overlain by a soft (Fig. 5b) or stiff (Fig.5c) non-Newtonian
viscous material was detached above a rigid base. Slip along the rigid
base and along the ramp caused the competent plastilina hangingwall to
be driven into the overlying, less competent layer. The soft overburden
material thinned above the ramp anticline and thickened in the area surrounding
the anticline (Fig. 5b). In this model, because the base was rigid there
was a physical continuity between the ramp and the flat gliding horizon,
movement was accommdated in the manner of fault bend folding. Moreover,
the load imposed by the advancing hangingwall created a footwall syncline.
By comparison,
when the competent plastina layer was overlain by stiff ductile strata
(Fig. 5c), the overthrust was small compared to the total shortening of
the stratigraphic package. The stiff overburden material acted as a more
or less rigid lid hindering forwards displacement along the ramp surface.
In consequence the initial ramp became modified to a listric shape with
deformation. In these two-layer models isostatic adjustment was not aided
by a density instability because the overlying materials were less dense
than the hangingwall/footwall plastilina blocks.
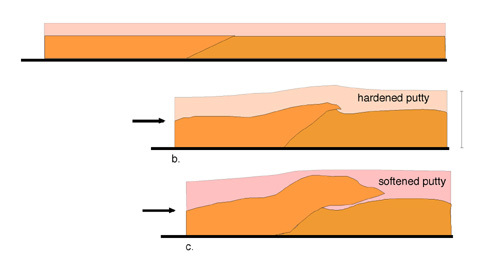 |
Figure 5.
a) Initial set-up of two-layer models detached above a rigid base,
while overlain by soft, respectively stiff ductile strata. b) and
c) represent the deformed models. (Select
image for enlargement) |
Ramp-flat
accommodation above ductile substrates
In other rheologically
stratified two-layer models (Fig.6a), the same materials as the previous
tests (Fig .5) were used, except that the hangingwall/footwall plastilina
blocks rested on ductile layers of various competence. In these models,
as compared to the previous ones (Fig. 5 b and c) there was no direct
physical continuity between the ramp surface and the flat basal decollement.
When the hangingwall/footwall plastilina blocks overthrusted a weak viscous
substratum (Fig.6b), the soft material was mobile enough to get injected
into the ramp during forwards transport of the hangingwall. In addition,
forward transport of the hangingwall downflexed the footwall which created
a shallower ramp along which movement of the hangingwall could easily
be accommodated. In these models adjustment of the plastilina hangingwall/footwall
blocks took place in the presence of buoyancy forces. The original 30§
ramp became rotated to near horizontal position during translation of
the hangingwall above the footwall. Such a two-layer model where a soft
substratum layer is transported from synclines to anticlinal core as the
upper competent layer ramps up is supported by geological data. For example,
salt and evaporite-cored anticlines beneath developing ramps are well
known in the Appalachian plateau (e.g. Wiltschko and Chapple, 1977) and
in the Jura (e.g. Suter, 1981; Jordan & Noack, 1992).
By comparison
when the competent material was underlain by a stiff ductile layer (Fig
6c), the initial straight ramp became wedge-shaped with deformation. In
addition, the overthrusting hangingwall accummulated higer layer parallel
shortening strain as compared to the previous model, with no or little
penetration of the ductile substratum beneath the ramp. The wedge fault
geometry seems to require low stiffness contrast between the competent
ramping layer and the surroundings.
Ramp-flat accommodation in embedded
ductile strata
Figures 7a-c illustrate a three-layer stratigraphic arrangement
where the plastilia single layer is sandwiched between ductile units of
various competence. The greater the contrast in stiffness, the higher
the tendency for the sandwiched competent member to develop buckle folds
rather than migrate forward in ramp-flat thrust style (Fig. 7b). In other
words, the induced ramp had little or no effect in guiding the subsequent
development of the overall structure. Rather. the competent layer buckled
and the adjacent soft matrix merely responded to the deflecting stiff
layer by offering an overall resistance to its deflection (Fig.7 b). Wavelength
selection of the stiff member was largely a function of the thickness
ratio and viscosity contrast between the stiff member and the softer matrix.
The soft matrix thickened beneath growing antiforms and thinned beneath
synforms, controlled by the folding instability of the competent plastilina
member.
In contrast,
the matrix materials was stiff, possessing yield strength. This provided
reduced stiffness contrast with the competent embedded member, the initial
straight ramp changed shape to a wedge fault, where a footwall synform
mirrored the hangingwall antiform (Fig. 7c), in the manner suggested by
Ramsay (1992) for the Kimmeridge model. The slip-dependent stretching
along the fault surface most likely determined the overall geometry of
the wedge fault (Fig.7c).
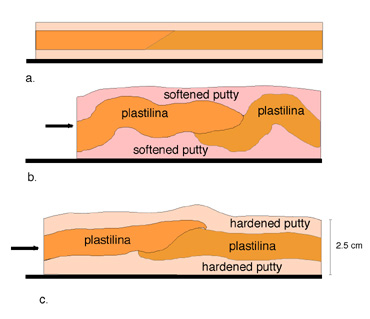 |
Figure 7.
a) Initial set-up of three-layer models where the competent single
layer is sandwiched between soft (b) and stiff (c) ductile strata.
(Select image
for enlargement) |
|