Introduction
The Semail ophiolite of Oman is perhaps the most intensely studied ophiolite
in the world and the presence of unusual silica, iron oxide/hydroxide
and carbonate hydrothermal alteration within it has long been known
(Glennie et al., 1974; Stanger, 1985; Villey et al., 1986). There has
been only one substantial study of this alteration (Stanger, 1985) despite
the fact that in other ophiolite bodies (e.g. Morocco, Saudi Arabia)
similar alteration is associated with potentially economic gold concentrations
(Buisson & LeBlanc, 1985 & 1987). In this paper, we present
new field observations, new mineralogical and geochemical data and geochemical
models of water-serpentinite interaction. We present a preliminary hypothesis
for the formation of this hydrothermal alteration and sub-economic platinum
mineralization, involving high heat flow associated with post-obduction
Tertiary faulting and magmatism and circulation of oxidised groundwaters
similar to those present today. Relatively Pt and Pd depleted chromite
pods are proposed as a possible source of these elements.
Previous
Work
Glennie et al. (1974) first mapped the silicified (“listwaenite”)
portion of the Semail ophiolite of Oman, as the Amqat lithostratigraphic
unit. Stanger (1985) noted that the Amqat unit is within the basal serpentinite,
sometimes being part of a basal thrust. He inferred that silicification
was spatially and temporally associated with Palaeogene fault movement.
Stangers’ work also suggested that the silica and iron oxides
replaced serpentinised ultramafic as evidenced by the presence of (presumably
residual) chromite and similar amounts of Al, Fe and Cr in serpentinite
and silicified serpentinite.
Elsewhere,
hydrothermally altered ophiolite (listwaenite) of Saudi Arabia, hosts
ancient gold workings at the margin of serpentinised ultramafic rock
masses. Hydrothermal alteration phases include talc, dolomite, magnesite,
magnesian chlorite and fuchsite, while chromite is a relict, metastable
phase inherited from the precursor rock (Buisson & LeBlanc, 1987).
Economic gold (> 1 ppm) occurs in pyrite- and gersdorffite-rich zones
and in late quartz veins, and has been observed at the margins of pyrite
crystals (Buisson & LeBlanc, 1987).
Another
example of precious metal associated with altered ophiolite is the Bou
Azzer ophiolite in Morocco (Buisson & LeBlanc, 1985 & 1987).
Small cobalt orebodies (now mined out) consisted of arsenide minerals
(e.g. skutterudite) in a calcite-dolomite gangue with hematite, magnetite
and quartz. About 5 tonnes of by-product gold was recovered. Elevated
gold (1 – 10 ppm) occurred in rocks containing high levels of
pyrite and cobalt arsenide or late pyrite and arsenopyrite-bearing quartz
veins (Buisson & LeBlanc, 1985 & 1987). Gold-rich rocks also
contain high levels of Sb, Bi and Ag. The contact between ore and serpentinite
was marked by a distinct talc-serpentine-carbonate zone (listwaenite)
and Mg-chlorite and serpentine become more abundant with proximity to
unaltered serpentinite, suggesting hydrothermal zonation.
Buisson & LeBlanc (1987) have argued that the gold was deposited
from modified CO2-rich seawater that
circulated through the ophiolite during serpentinisation. They cite
fluid inclusion evidence for temperatures of 150 to 250°C. Gold
was inferred to have been introduced as a sulphide or arsenide complex
and precipitated due to pH change and/or change in oxidation state that
resulted from interaction of hydrothermal fluid and carbonate rocks.
Gold is inferred to have been derived from magnetite contained within
the serpentinite.
This
model contrasts with Stanger’s (1985) proposed mechanism for the
formation of the Amqat “listwaenite” and associated carbonate-rich
rocks. He proposed an early serpentinization driven by seawater ingress
into the ophiolite. The seawater was regarded as a source of arsenic.
Formation of the Amqat “listwaenite” was however ascribed
to circulation of slightly acidic and CO2-rich
groundwater at less than 50°C.
Hopkinson (2001) provided an unusual explanation for the “listewaenite”
involving salt diapirism through the ophiolite. The evidence for diapirism
is, however, limited to the presence of rare salt-rich rocks in a fault
zone at Qantab. A plausible alternative explanation is that the salt
is derived from localized evaporation of contemporary groundwaters.
Geological
Framework
Four Main Tectonostratigraphic Units
The geology of the study area is shown as figure 1, which is based on
the mapping of Villey et al. (1986), as is the account of the regional
geology given below. Four major tectono-stratigraphic units can be differentiated.
The oldest rocks in the area, referred to herein as basement, are autochthonous
Ordovician to Cretaceous carbonate and clastic sedimentary rocks, summarized
in Table 1. Overall the rocks are red, yellow or mauve in colour. Pseudomorphs
after gypsum have been noted, but appear to be uncommon. The Saiq Formation
is conspicuously darker, and yields H2S
when struck with a hammer. Basaltic and andesitic volcanic rocks form
a small part of the overall basement sequence. Pyroxene phenocrysts
are preserved but the ground mass is commonly altered to an assemblage
of epidote, chlorite and Ti-oxide of uncertain age.
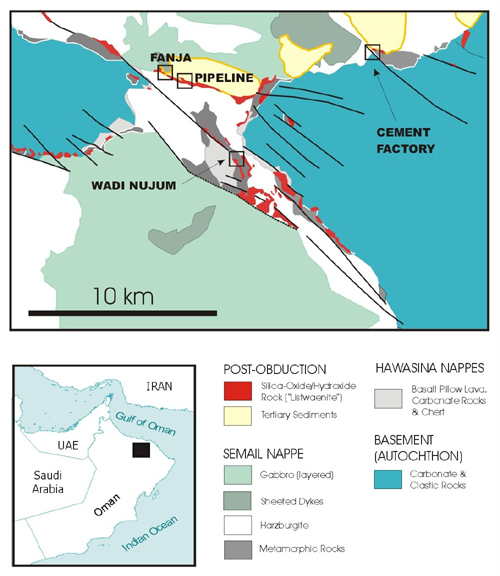 |
Figure
1. Geology of the Fanjah area, showing the distribution
of “listwaenite” simplified after Villey et al. (1986).
Quaternary deposits related mainly to wadis are not shown. |
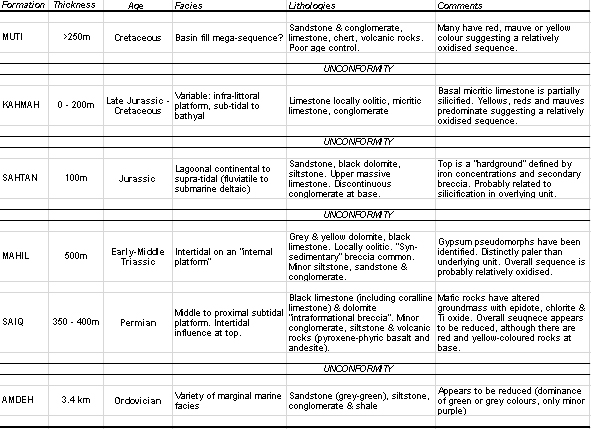 |
Table
1: Stratigraphy and lithologies of the pre-obduction autochthonous
basement (compiled from descriptions in Villey et al., 1986). |
Overlying
the basement rocks are autochthonous rocks of the Permian Al Jil Formation,
part of the Hawasina Nappes. These include alkalic basaltic to andesitic
lavas, shale, conglomerate, limestone radiolarian chert and sandstone.
Primary magmatic minerals include plagioclase, clinopyroxene and various
opaque minerals. Magmatic plagioclase has been replaced by albite and
carbonate and chlorite are common secondary phases. The chemical composition
of the lavas suggests formation in a rift environment (Cotton et al.,
2001).
The next major tectono-stratigraphic unit is the Semail nappe consisting
of ophiolitic harzburgite, separated by a discontinuous dunite layer
from overlying layered gabbro and a sheeted dyke unit of dolerite dykes
with chilled margins. These rocks were tectonically emplaced over the
basement during obduction in the Cretaceous (e.g. Hanna, 1992). Superimposition
of the ophiolite sequence on underlying sediments created quartzite,
garnetiferous muscovite schist, chlorite schist and amphibolite collectively
known as the metamorphic sole.
About
90% of the “mantle” sequence has undergone serpentinisation
of intensity varying between 55 and 85% by volume (Stanger, 1985). Veins
of carbonate (mainly magnesite) are almost ubiquitous (Stanger, 1984).
A range of Ni minerals has been described including acicular niccolite,
annabergite, maucherite, Ni-nontronite and Ni-montmorillonite (Haynes,
2001; Stanger, 1985; Hopkinson, 2002). Another alteration type is rodingite,
found in faults and joints, consisting of hydrogrossular, prehnite and
zoisite.
Overlying
gabbro also displays evidence for post-intrusion hydrothermal alteration,
namely the presence of tremolite-actinolite, epidote, chlorite, sphene,
prehnite, talc and magnetite and locally sulphide minerals (e.g. Nehlig
& Juteau, 1988). The age (or ages) of this widespread and locally
intense alteration, however, remains uncertain.
Post-obduction
cover rocks vary in age from Late Cretaceous to Quaternary and consist
of conglomerate, limestone and sandstone unconformably overlying all
older units. They were deposited in environments that range from marginal
marine to inner shelf (e.g. Racey, 1994). The Tertiary rocks are unmetamorphosed,
but in the vicinity of the cement factory (Fig. 1) show varying degrees
of silicification, and iron metasomatism and up to 100 ppb gold has
been recorded (Haynes, 2001). Locally, harzburgite faulted against the
Tertiary rocks has been altered to an assemblage of Ni-montmorillonite
and halloysite (Haynes, 2001).