The Monte Amiata: a Quaternary “hybrid” volcano
The Monte Amiata volcano, Late Pleistocene in age, is located in Southern Tuscany, well within the area of the Tuscan Magmatic Province (Fig. 11). The Monte Amiata volcano is a small linear volcano that produced few lava flows, domes and dome collapse (Fig. 13) with a high-K calc-alkaline to shoshonitic character (Fig. 14). Its chronology relies on several K/Ar and Fission Tracks data that cover the whole volcanic activity (Evernden & Curtis, 1965; Bigazzi et al., 1981; Pasquaré et al., 1983; Cadeaux & Pinti, 2009), and few 40Ar-39Ar data on the oldest products (Laurenzi & Villa, 1991; Barberi et al., 1994). The initial known activity start at about 300 ka (Barberi et al., 1994; Cadeaux & Pinti, 2009), hence all older ages reported in literature are problematic. The end of Amiata volcanic activity is quite undefined: Bigazzi et al. (1981) gave ages around 200 ka for the final lava domes, but Cadoux & Pinti (2009) dispute that conclusion and affirm that sanidine ages are “magmatic ages” and not eruption ages, leading to uncertain age assignment for all products. In spite of disputes on the time length of Amiata activity, this volcano is much younger of any other volcanic center belonging to the Tuscan Magmatic rocks (leucite-free ultrapotassic rocks) and well within the time span of Roman volcanic rocks (lecite-bearing ultrapotassic rocks).
Figure 13. Geological sketch map of the Monte Amiata volcano a hybrid volcanic center transitional between Tuscan and Roman Magmatic provinces
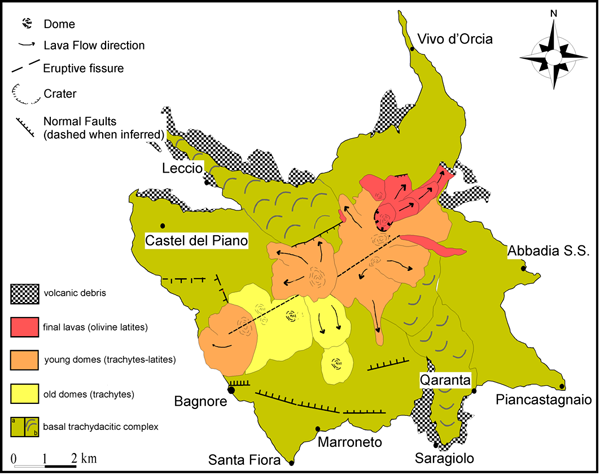
Geological sketch map of the Monte Amiata linear volcano. Redrawn after Ferrari et al. (1996).
The Monte Amiata volcanic rocks range from Two-Pyroxene trachydacites to olivine-bearing, orthopyroxene-free olivine latite to trachyte (Fig. 14a) (Ferrari et al., 1996). The oldest products belonging to the Basal trachytic complex are made up of biotite, plagioclase, sanidine, clinopyroxene and orthopyroxene, amphibole, Ti-magnetite and rarely by interstitial quartz (van Bergen, 1984; Ferrari et al., 1996). The youngest ones have a slightly bimodal composition with early emplacement of trachytic to latititic lavas (Fig. 14a), in the form of exogenous lava domes and short massive lava flows, and the final emplacement of olivine-latitic to shoshonitic lava flows (Fig. 14a). In addition Monte Amiata volcanic rocks are characterised by the occurrence of abundant magmatic enclaves and metasedimentary xenoliths ranging from centimetre to decimetre size. Metasedimentary xenoliths, ranging from angular to irregularly-rounded, are mostly flattened in shape and predominate in Basal Trachydacitic rocks. Fine-grained magmatic enclaves have ellipsoidal shape with cuspidate margins convex toward the hosts. The amount of fine-grained magmatic enclaves increases with decreasing ages of host rocks, from Basal Trachytic Complex to Final Lavas. Fine-grained magmatic enclaves range from porphyritic to aphyric and invariably display chilled margin texture, ranging in compositions from trachybasaltic to shoshonitic and latitic. Petrographic and compositional characteristics of fine-grained magmatic enclaves suggest that they were molten at the moment of the inclusion by the host trachytic magma. Their characteristics are strongly suggestive for the occurrence of interaction between different types of magmas prior to eruption (Ferrari et al., 1996; Cadoux & Pinti, 2009). This hypothesis is also supported by the occurrence of disequilibria texture among the mineral assemblages of the dome complex (intermediate volcanic activity) (Fig. 3e).
From a petrological point of view the Monte Amiata volcanic rocks were produced by the crystallization of strongly differentiated shoshonitic magma with trachytic to trachydacitic compositions (Ferrari et al., 1996; Cadoux & Pinti, 2009; Conticelli et al., 2009c). This magma produced the early eruption of the basal trachytic complex and of the trachytic to latitic dome complex (Fig. 14a). Conticelli et al. (2009c, 2010b) suggested that the early Monte Amiata magma derived by the fractional crystallization plus crustal contamination starting from a parental magma similar in composition to the Radicofani shoshonitic trachybasalt. The intermediate and final eruptions of the Monte Amiata have been produced by the mingling between this extremely differentiated high silica magma and ultrapotassic silica-undersaturated magmas (Roman) (van Bergen et al., 1983). The arrival in the shallow level magmatic reservoir of newly formed leucite-bearing hot magma from the source region triggered Monte Amiata eruptions. Mafic enclaves represent the evidence of the mingling between leucite-bearing Roman magmas and the relic high-K calc-alkaline magmas of the previous Tuscan episode. Leucite crystallization from Roman magma has been suppressed by the strong silica activity of the stagnant differentiated Tuscan magmas, crystallizing in the magma reservoir at shallow depth. These processes well explain the characteristic composition of the Monte Amiata rocks and the very young time span of volcanic activity, suggesting that the Monte Amiata is neither a Tuscan nor a Roman volcano, but just a “hybrid” volcano due to the interaction between leucite-free and -bearing potassic to ultrapotassic magmas (Conticelli et al., 2009c).
Figure 14. Classification and incompatible trace element characteristics of Quaternary Monte Amiata volcanic rocks
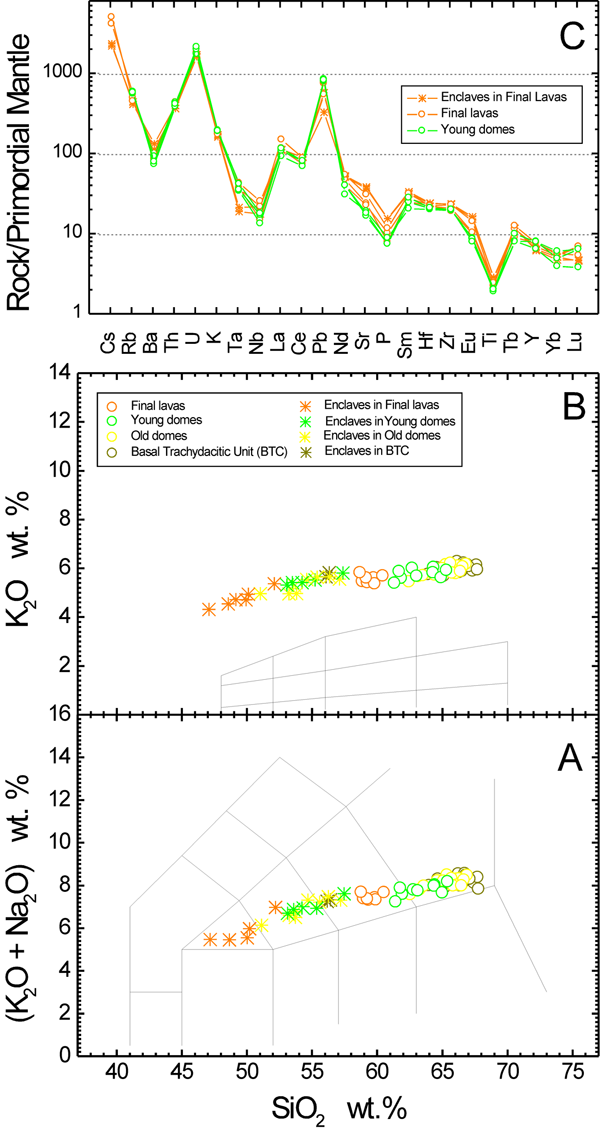
Classification and geochemical characteristics of the Monte Amiata volcanic rocks and mafic enclaves. A) Total Alkali-Silica (TAS) classification diagram (Le Maitre, 2002). B) K2O wt.% vs. SiO2 wt.% classification diagram with reported the grid for orogenic volcanic rock suites (Peccerillo & Taylor, 1976). C) Incompatible trace element patterns for mafic volcanic rocks normalised to the primordial mantle values of Sun & McDonough (1989). Data from Ferrari et al. (1996), and authors’ unpublished data.
The mingling hypothesis is also testified by the incompatible trace element distribution shown in figure 14c. Indeed mafic Final Lavas display normalized patterns very similar to those of the Tuscan rocks, with troughs at Ba, Ta, Nb, P, and Ti, and peaks at Cs, U, and Pb. The strong peak at U with extremely high U/Th ratios is peculiarly different, and it is much higher than in the rest of the Tuscan rocks (Fig. 14c). Incompatible trace element patterns of mafic enclaves differ greatly from those of the lavas in terms of normalized Ta/Nb, Nd/Sr, and Zr/Hf, although no differences have been observed among U/Th ratios (Fig. 14c).