The IPB volcanism displays a bimodal distribution with no significant amounts of intermediate rocks and felsic rocks predominating over mafic rocks (Mitjavilla et al., 1997; Munhá, 1983). As shown by Rosa, D. et al., (2004), most intermediate compositions are apparent and reflect silica and alkali mobility and subsequent compositional scatter on major element diagrams. This silica and alkali mobility was focused along fracture networks and within the matrices of hyaloclastic breccias. This is significant for the geotectonic classification of the volcanism, as arc settings are typically dominated by intermediate rocks (andesites), while volcanism generated in extensional settings is generally bimodal.
The felsic rocks present are of calcalkaline affinity. They are represented by pumice-rich facies and coherent or brecciated porphyritic facies. In some areas, only the porphyritic facies has been identified (Albernoa area), whereas in the areas where both facies are present, porphyritic facies can erupt after the pumice-rich facies (Neves-Corvo) or the two facies can alternate (Serra Branca). The felsic rocks have phenocryst populations and compositions corresponding from rhyodacitic to rhyolitic compositions. This is confirmed through their modal classification on QAP space (Streckeisen, 1978). The narrow range of compositions results from some fractional crystallization, namely of feldspar, as indicated by progressive increase in the whole-rock negative Eu anomaly with fractionation.
The high-field-strength elements (HFSE) systematics of the IPB felsic rocks is variable and their use for the classification of the IPB tectonic setting needs to be carefully addressed. Studies of felsic rocks from Albernoa have shown that these rocks are misclassified when plotted on the Winchester and Floyd (1977) diagram, where they plot as andesites (Figure 9). However, at Serra Branca (Figure 9), the Winchester and Floyd (1977) diagram provides consistent results. The problematic classification of the Albernoa samples is partially caused by their relatively high Ti contents. However, according to Rosa, D. et al (2004), it is also due to their anomalously low Zr contents. In fact, other HFSE are anomalously low at this location. The anomalously low HFSE contents are possibly caused by a decreased solubility of the refractory phases in which these elements reside, during low temperature crustal fusion. This same process has been suggested by Piercey et al. (2001) and Lentz (1999) for felsic rocks from the Finlayson Lake District, Yukon, and Bathurst, New Brunswick, respectively.
Figure 9. Discrimination diagram
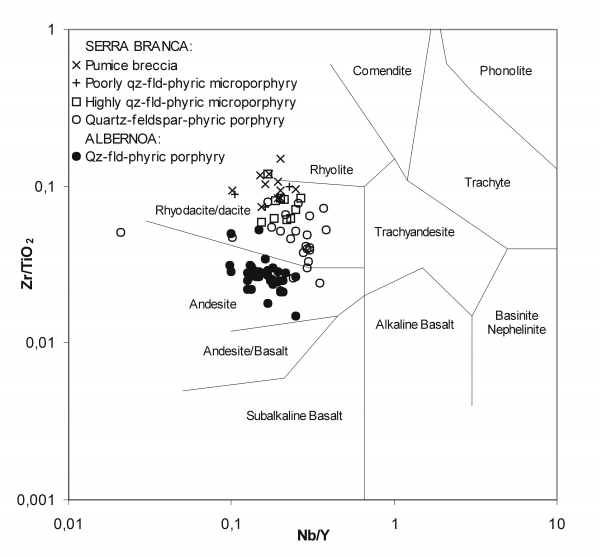
Winchester and Floyd (1977) discrimination diagram, displaying the (mis)classification of felsic rocks from Albernoa and Serra Branca.
Rosa, D. et al (2005) looked into the temperature conditions for crustal fusion, determining zircon and monazite saturation temperatures (Watson and Harrison, 1983; Montel, 1993) for one area with anomalously low and one area with normal HFSE contents; Albernoa and Serra Branca, respectively. The zircon and monazite saturation temperatures generally display good agreement between them, confirming their reliability. Outliers, which depart from the TZr=TLREE line, are accounted for by considering that they correspond to samples with significant amounts of inherited zircon and/or monazite, or for which not enough time elapsed for REE saturation before melt extraction. The obtained temperatures are interpreted as being maximum temperatures of crustal fusion and, since there is only limited fractionation, they also provide an upper limit to the emplacement temperature. The zircon thermometer indicates temperatures for the magmas that yielded the porphyritic rocks averaging approximately 780ºC at Albernoa and 820ºC at Serra Branca. At Serra Branca, another magma, which generated the pumice-rich facies, was emplaced at approximately 770ºC, as indicated by the zircon saturation temperature (Rosa, D. et al., 2005).
The zircon and monazite saturation temperatures indicate that magmas at the studied sites were generated at different temperatures, which has exploration implications. At Serra Branca, two different magmas were emplaced, one at a significantly higher and the other at a slightly lower temperature than the temperature recorded at Albernoa. Higher magma temperatures may help develop and sustain hydrothermal convection cells and be a prerequisite for the generation of VMS deposits. Also, in this geological setting, higher temperature fusion occurs under lower pressures, i.e., under shallower conditions, again favoring hydrothermal circulation and VMS formation (Hart et al, 2004). This may explain why Albernoa appears to be barren, while Serra Branca has a gossan, despite sub-economic (Rosa, D. et al, 2005).
If the HFSE concentrations can be anomalously low, the geotectonic settings indicated by diagrams that use these elements, such as the tectonic diagrams of Pearce et al. (1984), should be considered carefully. As pointed out by Rosa, D. et al. (2004), apparent arc signatures in Albernoa samples are the result of low temperature crustal fusion. In fact, considering that Ta, Nb, Yb and Y, similarly to Zr, would have been two or three times more concentrated if fusion had occurred under higher temperature conditions, the studied rocks would plot within or close to the within-plate granites field, instead of the volcanic-arc granites field (Rosa et al, 2004). This is compatible with models invoking partial melting of crustal rocks as a source of silicic magmas in the IPB, proposed by Munhá (1983), Mitjavila et al. (1997) and Thiéblemont et al. (1998).
In the IPB, the mafic rocks are basaltic dykes and flows of tholeiitic and, rarely, alkaline affinity. In the Nb-Zr-Y (Meschede, 1986) and Th-Ta-Hf (Wood, 1980) diagrams, the tholeiitic samples plot in or very close to the VAB field, while the alkaline samples plot as WPB (Rosa et al, 2004). Similarly, in the Zr-Y-Ti (Pearce and Cann, 1973) diagram, in which only tholeiitic rocks can be plotted, the samples plot on or very close to the VAB field (Rosa et al, 2004). However, these tholeiitic samples plot within the MORB+FB field and not on the island-arc field of the Shervais’ (1982) Ti-V diagram, considered to be less sensitive to crustal interaction (Pearce, 1996). This is an indication that the Th enrichment and coupled Nb depletion, evident in the Nb-Zr-Y and Th-Ta-Hf diagrams, likely result from the assimilation of continental crust rather than from a true subduction component (Pearce, 1996). Similarly, the Ti and Y depletion in the Zr-Y-Ti diagram is probably due to the magma interaction with relatively Ti- and Y-poor and Zr-rich continental crust. It is not completely clear what the original magma type would have been before assimilation of such continental crust and the described acquisition of an apparent arc signature. Yet the original magma may have had a MORB source, as Mitjavila et al. (1997) argue; a WPB source, in which case it could be represented by the alkaline basalts; or may have had a transitional source between the two.
The developed bimodal volcanism, with possible WPB/MORB transitions and superimposed crustal assimilation is characteristic of magmatism in attenuated continental lithosphere settings, such as the magmatism developed in continental crust by local extensional tectonics. This is compatible with the model proposed by Silva et al. (1990), Quesada (1991) and Solomon and Quesada (2003). In this model, an oblique collision between the South Portuguese and the Ossa Morena plates favoured strike-slip tectonics within the continental crust of the South Portuguese plate. The strike-slip tectonics would have favoured the opening of pull-apart basins where mantle upwelling and subsequent high heat flow would lead to volcanism with the characteristics described above. A similar conclusion was drawn by Mitjavila et al. (1997), who in addition to general geochemical data, also presented isotope data.