The presence of excess 40Ar has been recognised especially in high-pressure metamorphic rocks (e.g. Arnoud and Kelley, 1995; Inger, 1996; Scaillet, 1996; Ruffet, 1997; Sherlock and Kelley, 2002). Under the assumption of mobility of argon in a crystal lattice by volume diffusion, excess 40Ar is expected to produce age spectra that are defined at the lower limit by a potentially real geological age while the upper limit is unconstrained and tends to go to values without any geological meaning (e.g. Pankhurst et al., 1973; Harrison and McDougall, 1981). Diffusion gradients with high apparent ages at the grain boundaries, decreasing toward the crystal cores are typical for excess argon (Reddy et al., 1996; Sherlock and Kelley, 2002). Grains containing inherited argon on the other hand, are more likely to show the reverse pattern with higher ages found in the cores of the grains.
Different scenarios for incorporating excess Ar, briefly described as follows, have been proposed (e.g. Arnoud and Kelley, 1995).
-Incorporation of a large quantity of excess argon during HP peak metamorphism, followed by a partial redistribution of argon during retrogression. This process would produce a range of ages in different units as a result of the non-uniform later partial redistribution of Ar during retrogression.
-Incorporation of argon during the HP peak, but also during the retrogression path. This process, as well, would create a wide range of ages due to the different retrogression paths experienced by different Alpine units.
Examples of excessively high 40Ar/39Ar spot ages are discussed by Arnaud and Kelley (1995), but on the whole, appear to be rare for the Western Alpine domain. The rock units exposed in the Western Alps today seem to be characterised mostly by ages that do not exceed the inferred protolith age of the rock units exposed.
With the new age data presented in this paper we add to the existing picture in that we demonstrate that over a period of several tens of millions of years before the present, the detritus shed by erosion from the Western Alps is characterised by the same set of Cretaceous age groups. This new finding is surprising as it means that at the time of, and very shortly after, the Eocene metamorphism, the detritus is already characterized by these Cretaceous ages. However, the source of the TPB sediments has changed through time and included different Alpine units. Therefore, it is not realistic to think of a uniform reservoir for different sources. Further, the nature of diffusion, governed by transport in terms of exponential transport functions would predict age distributions that essentially would follow a Maxwell-Boltzman distribution (Figure 7). As transport is highly chaotic and dependent on the parameters of individual rock units (e.g. permeability, composition), the actual form of the Boltzman distribution in terms of average, median and skewness will depend critically upon local characteristics of individual rock units. The concentrations of excess argon in the grain boundary reservoir are predicted to be variable in space and time. However, maximum concentrations are unconstrained. Thus, it would be expected that excess argon would produce a range of ages that is constrained on the young side by the actual age of the rock, but on the high age side it is essentially open ended. In fact this is observed in cases where the presence of excess argon is undisputed such as the granulite terrains of Broken Hill (Harrison and McDougall, 1981) and south west Greenland (Pankhurst et al., 1973).
Our data are inconsistent with the excess argon model for pre-Eocene ages as they are showing clearly defined age groups that are essentially constant over extended periods of time. Thus we argue that they should be interpreted to be caused by the effects of inherited argon rather than the effects of excess argon. The subsequent Paleogene metamorphic history of the area (Reddy et al., 2003) has served to obscure the pattern of earlier ages in that in areas of more intense deformation and recrystallisation Eocene and Oligocene ages can be found (see also Scaillet et al., 1992). However, in those areas removed from the zones of intense deformation and recrystallisation, temperature alone during the Eocene and Oligocene was not enough to fully reset the age of existing minerals, and thus pre- Eocene ages survived. This must have been the case for those rocks that eroded mainly during the Miocene in which the effects of Paleogene metamorphism might have been negligible.
Figure 7. Maxwell-Boltzman distribution
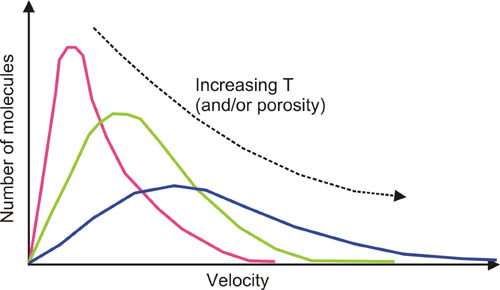
Maxwell-Boltzman distribution for the gas law (the plot of the number of molecules concentration versus the speed; as the temperature increases, this curve broadens and extends to higher speeds). In our case the shape of the curves will depend on different single parameters of rocks such as permeability and composition.