Discussion
General discussion
Slab deformation
Asymmetrical retreat of a subducting slab requires the least work to
deform the slab, simply because it does not involve extensive internal
slab deformation. This would be the case in other types of rollback,
such as radial rollback. To allow for asymmetrical slab retreat, the
only permanent large-scale deformation the subducting plate has to undergo
is the formation of a lithospheric-scale vertical tear on one side of
the slab segment (Fig. 2). Examples of such tears can be observed in
several arcs such as the Tonga Arc and the Ryukyu Arc. The formation
of a tear could be facilitated by the presence of a pre-existing weak
zone in the subducting lithosphere such as a transform fault, striking
at high angle to the arc. The presence of a vertical tear at one cusp
of the arc would further facilitate asymmetrical rollback, since it
would allow lateral asthenosphere flow around the slab in addition to
flow underneath the slab tip (Fig. 2c). A continuity of the slab across
the other cusp would retard rollback of the slab in the proximity of
this cusp, since the asthenosphere would only be allowed to flow underneath
the slab tip. This process would then amplify the entire process of
asymmetrical rollback and back-arc opening. Asymmetrical rollback could
also be facilitated by a density polarity in the subducting lithosphere,
parallel to the trench axis. This density polarity could result from
a variation in age of the subducting slab parallel to the trench or
the presence of buoyant features on the subducting plate. With such
a slab configuration, the denser part would have a tendency to roll
back faster compared to the lighter part, thus promoting asymmetrical
rollback.
Overriding
plate segmentation
In natural arc systems, slab retreat does not result in the separation
between the overriding and subducting plates along the trench. Instead,
the overriding plate fails at some distance from the trench. In ocean-ocean
subduction settings this often occurs along the volcanic arc, resulting
in the formation of a remnant and active volcanic arc, separated by
a back-arc basin [Karig, 1970, 1971a,b; Kobayashi and Nakada, 1979].
Examples include the Tonga-Kermadec Ridge, which was split from the
Lau-Colville Ridge during formation of the Lau-Havre Basin. Another
example is the Izu-Bonin Arc in the Western Pacific, which was split
from the Palau-Kyushu Ridge during formation of the Parece-Vela Basin.
More recently, the active Mariana Ridge was split from the inactive
West Mariana Ridge during formation of the Mariana Trough. In ocean-continent
subduction settings, back-arc deformation is more diffuse. However,
in a more advanced stage of deformation, retreat of the hinge-line can
result in the segmentation of the overriding plate, where a segment
is being separated from the continent. One example is the Kuril Arc,
which separated from the Okhotsk microplate during opening of the Kuril
Basin [Maeda, 1990]. Another example is the Ryukyu Arc, which is presently
being separated from East China, accompanied by extension in the Okinawa
Trough [Uyeda, 1977]. Other examples include the Japan Arc [Uyeda and
Miashiro, 1974] and the South Shetland Arc [Lawver, 1995].
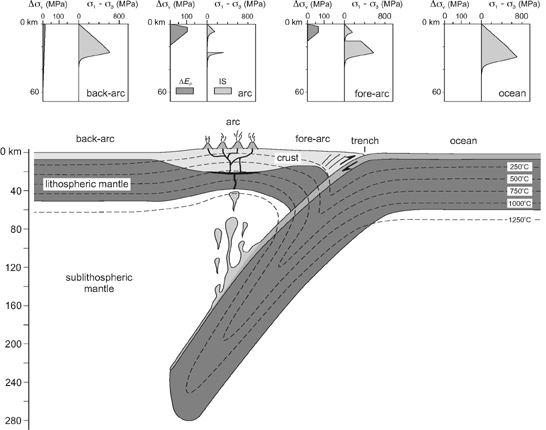 |
Figure 6. Schematic
sketch of ocean-ocean subduction system with inactive back-arc,
demonstrating the mechanical weakness and high potential energy
(with respect to the subducting lithosphere) of the arc compared
to the fore-arc region and the inactive back-arc region, implying
that during rollback of the subducting slab, the overriding plate
is most likely to break up along the arc. ÐEP =
potential energy difference; IS = integrated strength. |
A number of arguments
can be given for the reason why the overriding plate separates at the
arc and not at the trench. First of all, the strength of the overriding
plate is at its smallest at the arc due to thermal weakening and its
relatively thick crust (Fig. 6). Also, the arc has the highest potential
energy, further promoting separation along the arc. This excess potential
energy results in a net push from the arc towards the overriding plate,
which will therefore not allow separation between the overriding and
subducting plate during rollback. Injection of asthenosphere material
along the subduction boundary does not occur because of this push from
the arc region to the subducting plate, the lithostatic pressure exerted
by the overlying mass on the boundary and the net downward drag of material
along the subduction boundary due to subduction of the slab.
|
Figure 7. Schematic
sketches illustrating extension observed in experiments and the
formation of a ridge (i.e. arc) along the retreating door. In most
experiments the brittle strength (BS) of a column of lithosphere
is smaller than the buoyancy force (BF = potential energy difference
(DEP) between column of glass beads and column of glucose syrup).
This results in a gradual spreading of the overriding lithosphere
during retreat of the door, where the spreading layer remains connected
with the retreating door. (a) Initial stage. (b) Initial extension
close to the retreating boundary. (c) Continuing retreat leads to
a migration of the deformation front. (d) Formation of a principal
zone of extension (PZE = zone absorbing most of the extension) and
a ridge (arc), which is attached to the retreating door. The location
of the PZE and arc could be related to the boudinage of the lithosphere
due to retreat of the door, where the silicone layer is not allowed
to neck at the contact zone with the door, because this would result
in high vertical shear stresses. |
In most experiments,
no separation between the overriding plate and the retreating door occurred
as well, even though the overriding plate was homogeneous throughout.
This could be explained by the fact that in most experiments the buoyancy
force was greater than the brittle strength of the overriding lithosphere,
resulting in spreading of the overriding lithosphere over the glucose
syrup in the space it was allowed to by the retreating door (Fig. 7).
In an advanced stage of deformation, a ridge segment (i.e. arc) developed
in between the retreating door and the principal zone of extension (PZE
= a zone which developed in an advanced stage of deformation and subsequently
absorbed most or all of the extension during continuing deformation).
The location of the PZE and the ridge (arc) could be related to boudinage
of the lithosphere due to retreat of the door, where the silicone layer
did not favour necking at the contact zone with the door, because this
would result in high vertical shear stresses along the contact zone.
Thus, it can be concluded that from pure mechanical reasons and even
in a homogeneous overriding plate, rollback promotes extension to be
localised at some distance from the subduction interface.
|
Figure 8. Schematic
sketches to explain extension observed in experiment 5 (Fig. 4b)
and experiment 7 (Fig. 4a), where the integrated strength (IS) of
a column of glass beads is much greater than the buoyancy force
(BF = potential energy difference (ÐEP)) between
column of glass beads and column of glucose syrup). (a) Initial
stage. (b) Incremental retreat of the door over a distance dx.
ÐEP between column of glass beads and the gap created
by the retreat of the door is greater than the integrated strength
of a column of glass beads. (c) Collapse of the glass beads layer
towards retreating door. (d) Continued retreat of the door may lead
to separation of door from glass beads (e.g. experiment 5). |
An interesting question
that arises from experiment 5 (and also experiment 5 and 9) is why the
overriding plate did not immediately separate from the retreating boundary,
but only did so after some considerable deformation of the overriding
plate (Fig. 4a,b). One would not expect any deformation in the overriding
lithosphere due to spreading, since the buoyancy force (between a column
of glass beads lithosphere and a column of glucose syrup asthenosphere)
in this experiment is a factor of ~ 8-9 smaller (see table 2) than the
integrated strength of the overriding lithosphere. Evidently, the retreating
boundary can transmit deviatoric tensional stresses to the overriding
lithosphere, without there being any significant adhesion between the
glass beads and the retreating door. An explanation for this behaviour,
based on the results of experiment 5, is sketched in Fig. 8. From an
initial stage (Fig. 8a), retreat of the door over a short distance dx
leads to the formation of a lithospheric scale gap (Fig. 8b), which
will not immediately be filled with glucose syrup. This will result
in a large potential energy difference between the overriding lithosphere
and the gap, leading to the failure and collapse of the overriding lithosphere
into the gap (Fig. 8c). Extension continues until at some stage the
overriding lithosphere separates from the door (Fig. 8d).