Below, we will briefly describe two natural examples of arc - back-arc systems displaying a structural asymmetry. The first example is the Tonga Arc from the southwest Pacific and the second example is the Ryukyu Arc from the Philippine Sea.
The Tonga-Kermadec Arc system is located in the Southwest Pacific, where the Pacific oceanic lithosphere is subducting westwards along the Tonga-Kermadec Trench underneath the Indo-Australian plate (Figure 9). The Tonga arc is the northernmost half of the Tonga-Kermadec subduction zone, which is usually divided into two arc systems, based of the presence of the Louisville Aseismic Ridge, located on the subducting Pacific plate. From west to east, the overriding plate consists of four distinct zones (Figure 9):
(1) The South Fiji Basin, an Oligocene back-arc basin floured by oceanic crust [Weissel 1981].
(2) The Lau-Colville Ridge, which is a remnant volcanic ridge [Karig 1970].
(3) The Lau-Havre Basin, an active back-arc basin, which started extending at 7-6 Ma [Parson and Hawkins 1994]. Spreading started in the Lau Basin in the north at 5.5-4 Ma and has progressively migrated southward [Parson and Hawkins 1994]. At present the spreading ridge has reached as far as 24° S [Wiedicke and Collier 1993], which is the southern most part of the Lau Basin. The Havre Basin is still in its extensional phase [Gamble and Wright 1995]. The extension is propagating south-southwestward, with intra-continental extension in the Taupo Volcanic Zone in New Zealand.
(4) The Tonga-Kermadec Ridge, which is the active volcanic ridge.
Figure 9. Tonga Arc region
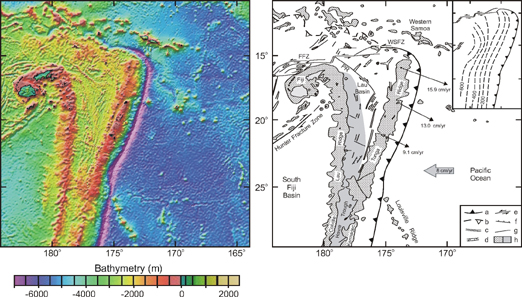
(a) Bathymetric map of the Tonga Arc region (from Smith and Sandwell [1997]). (b) Regional tectonic setting of (a) (compiled after Wiedicke and Collier, [1993], Wiedicke and Habler, [1993], Sager et al. [1994], Bevis et al. [1995], Pelletier et al. [1998] and Fujiwara et al. [2001]). GPS velocity (thin arrows) of Tonga Arc relative to Australian plate (which includes Fiji block and Lau Ridge) (from Bevis et al. [1995]). Thick arrow represents relative convergence between Australian and Pacific plate (from DeMets et al. [1994]). Isobaths of Wadati-Benioff zone are shown in inset (100 km contours). a, trench (triangles on upper plate side); b, remnant trench; c, spreading axis; d, graben/trough; e, strike-slip fault; f, normal fault; g, fault (undifferentiated); h, main morphostructural feature on ocean floor (left) and extended crust in back-arc basin (right) (modified from Parson and Hawkins [1994]). FFZ = Fiji Fracture Zone, PR = Peggy Ridge, WSFZ = Western Samoa Fracture Zone. (Click for enlargement)
Two models have been proposed to explain the wedge shaped geometry of the Lau Basin. In the first model the basin supposedly has progressively opened up from north to south (e.g. unzip model [Hawkins 1995]). The second model is the so-called scissors or windscreen wiper model, where the rate of opening of the basin increases northwards [Sager et al. 1994; Bevis et al. 1995]. A GPS survey of the Tonga Ridge has revealed that the rate of opening increases from south to north and that the Tonga Ridge shows little or no arc parallel deformation [Bevis et al. 1995], indicating that the latter model is most applicable. Paleomagnetic data indicates that the Tonga Ridge has rotated ~ 20° during opening of the Lau Basin, comparable to the amount suggested by the wedge-shaped geometry of the basin itself [Sager et al. 1994]. In the scissors model, the hinge point is defined by the Louisville Seamount chain located at the southern cusp of the arc. At the northern cusp of the arc, deformation is accommodated by the Western Samoa Fracture Zone. Here, the Tonga Arc ends abruptly and the strike of the trench rotates some 90° from north-northeast to west-northwest. At depth, this corresponds to the vertical limit of the Tonga slab at ~ 15°S (Figure 9), which seems to have formed due to tearing between the slab and the Pacific plate at the surface [e.g. Millen and Hamburger 1998]. This tear could have formed due to asymmetric rollback of the Tonga slab, as schematically sketched in Figure 2.
The Lau Basin has formed by two tectonic styles of extension. Initially, the western part of the basin formed by crustal extension and rifting, accompanied by magmatism that partly filled the rift basins [Hawkins 1995]. This was followed by a phase of seafloor spreading [Hawkins 1995] along spreading axes located more to the east. These spreading axes developed on rifts that started from a transform fault boundary [Hawkins 1995].
The basin structures can best be compared with the model results presented in Figure 4c, where extension is localised close to the retreating boundary. The model results show that initially extension is absorbed over a wider area, but is later accommodated entirely by a zone located just behind the rifted arc sliver. This is similar to the opening history of the Lau Basin. Furthermore, extensional structures in the basin are mainly parallel to the arc itself, as also observed in the model. This is most probably related to the early stage of opening of the basin. In a later stage of opening, one could expect arc-oblique and perpendicular spreading ridges to form near the hinge point (i.e. near the Louisville Ridge). For instance, this is observed in the North Fiji Basin, which has opened up by ~ 40-50° of rotation of the New Hebrides Arc [Musgrave and Firth 1999]. Here, rift axes and spreading ridges near the northwestern cusp of the arc (i.e. hinge point) are oriented at high angle to the arc but are oriented more parallel to the arc towards the southeast (Figure 1) [Schellart et al. 2002a].
The Ryukyu Arc is located along the South China active margin in an ocean-continent subduction setting. Here, the Philippine Sea plate is subducting northwestward along the Ryukyu Trench underneath the Ryukyu Arc (Figure 10). Two cusps define the lateral extent of the arc. The northeastern cusp is geographically linked to the Palau-Kyushu Ridge and the southwestern cusp to Taiwan. The Ryukyu Arc is flanked on the northwestern side by the Okinawa Trough, an active back-arc basin, which has started extending since the Late Miocene [Letouzey and Kimura 1985] and is at present still in a rifting stage. The Ryukyu Arc itself has also experienced deformation from the Late Miocene to Present, with arc parallel as well as arc perpendicular extension [Fournier et al. 2001]. The most recent stage of rifting (~ 1 Ma - Present [Miki 1995]) in the Okinawa Trough occurs within a series of en echelon left-stepping grabens trending NE-SW in the north and ENE-WSW in the south (Figure 10) [Sibuet et al. 1987]. In the north, the graben axes strike oblique to the main trend of the Okinawa Trough. The crustal thickness beneath the graben axis increases from 15-18 km in the southern part near Taiwan to 27-30 km in the northern part near Kyushu [Iwasaki et al. 1990; Sibuet et al. 1995].
Figure 10. Bathymetric map of the Ryukyu Arc
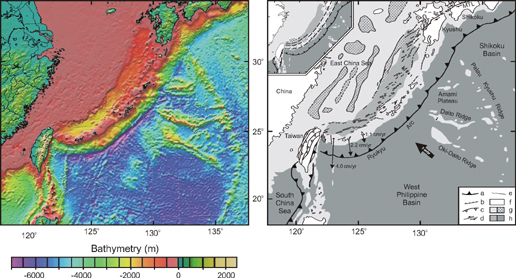
Bathymetric map of the Ryukyu Arc (a) Bathymetric map of the Ryukyu Arc and surrounding (from Smith an Sandwell [1997]). (b) Tectonic interpretation of (a) (compiled after Sibuet et al. [1987], Kamata and Kodama [1994] and Lallemand et al. [1999]. Horizontal GPS motions of the southern Ryukyu Arc with respect to South China (thin arrows) are from Imanishi et al. [1996] and Heki et al. [1996]. The relative motion of the Philippine Sea plate with respect to Eurasia (thick arrow) is from Seno et al. [1993]. Isobaths (dashed lines) of the Wadati-Benioff zone (for 50, 100, 150 and 200 km) are shown in the inset (from Eguchi and Uyeda [1983]). a, trench (triangles on upper plate); b, normal fault; c, thrust fault; d, strike-slip fault; e, fault (undifferentiated); f, land; g, continental shelf/morphological feature on ocean floor (left) and depression in East China Sea (right) (modified from Hsu et al. [2001]); h, Okinawa Trough (left) and continental slope/ocean floor (right). MTL = Median Tectonic Line. (Click for enlargement)
Several models have been proposed to explain the opening of the Okinawa Trough, such as: opening of the Okinawa Trough as a consequence of the collision in Taiwan [Letouzey and Kimura 1985]; opening in a pull-apart fashion due to India-Eurasia collision [Fournier et al. 2001]; rollback of the subducting Philippine Sea plate towards the SE [Viallon et al. 1986]. We suggest that the extension in the Okinawa Trough is best explained by rollback of the Philippine Sea plate towards the southwest. We propose that the most recent extension is the result of asymmetrical rollback, where the retreat rate increases from northeast to southwest, leading to asymmetrical extension in the overriding plate. Asymmetrical retreat could be explained by the presence of multiple buoyant features located on the subducting lithosphere along the northeastern part of the Ryukyu Trench (e.g. Palau-Kyushu Ridge, Amami Plateau, Daito Ridge, Oki-Daito Ridge). This would imply a higher average lithospheric density along the southwest of the trench compared to the northeast, resulting in a greater rollback velocity in the southwest. Also, a vertical tear in the slab in the southwest of the arc would facilitate the lateral flow of asthenosphere material around the slab edge from underneath the slab towards the slab corner to accommodate rollback (e.g. Figure 2c). This model could explain the en echelon pattern of the individual rift segments in the Okinawa Trough.
The analogue models also show that the obliquity of individual rift systems increases towards the hinge. On a smaller scale, axes of individual rift segments, which are part of large-scale rift systems, are normally oriented oblique to the rift system itself and become progressively more oblique to the rift system towards the hinge. This is also observed for rift segments in the Okinawa Trough. From comparison, experiments 8 and 4 (Figure 4c,e) show most resemblance with the Okinawa Trough. Experiment 4 show the development of a main extensional zone accommodating most of the extension (e.g. Okinawa Trough), located close to the retreating boundary with a narrow strip of material in between (i.e. comparable to the Ryukyu Arc). In the early stages of the experiment extension also occurs along individual rift segments located further away from the retreating boundary, similar to extension observed in the East China Sea (Figure 10). The extension in the north stopped after a through-going rift zone developed in experiment 4, similar to the extension in the East China Sea, which stopped after initiation of formation of the Okinawa Trough [Hsu et al. 2001].
Figure 11. Comparison between analogue results and structures
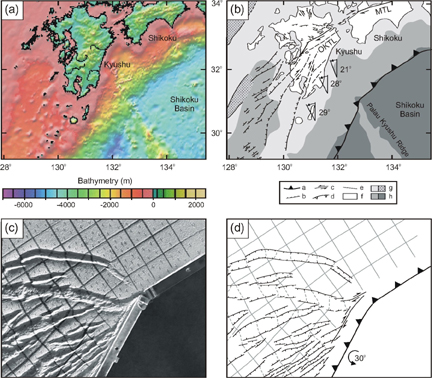
Comparison between analogue results of experiment 8 and structures in the overriding plate around the Palau-kyushu Ridge (from Smith an Sandwell [1997]). (a) Bathymetric map surrounding the northeastern cusp of the Ryukyu Arc. (b) Tectonic interpretation of (a) (compiled after Sibuet et al. [1987] and Kamata and Kodama [1994]. Paleomagnetic declinations from Kodama and Nakayama [1993] and Kodama et al. [1995]. (c) Results of experiment after 30° rotation. (d) Interpretation of (c). a, trench (triangles on upper plate); b, normal fault; c, thrust fault; d, strike-slip fault; e, fault (undifferentiated); f, land; g, continental shelf/morphological feature on ocean floor (left) and depression in East China Sea (right) (modified from Hsu et al. [2001]); h, Okinawa Trough (left) and continental slope/ocean floor (right). MTL = Median Tectonic Line; OKTL = Oita-Kumamoto Tectonic Line. (Click for enlargement)
Another interesting analogy between model and nature is the presence of the Oita-Kumamoto Tectonic Line (Figure 11a,b), a dextral strike-slip fault, which could be compared with the occurrence of dextral strike-slip faults near the hinge in most of the analogue experiments with a similar orientation compared to the trench (Figure 11c,d). In most tectonic interpretations the Median Tectonic Line, located to the east of the Oita-Kumamoto Tectonic Line, has been interpreted as resulting from strain partitioning in the overriding plate due to oblique subduction of the Philippine Sea plate [Tsukuda 1992; Kamata and Kodama 1994]. This interpretation could correct for the Median Tectonic Line sensu stricto. However, in the light of the experiments presented here, its westernmost extension outcropping on Kyushu Island (Oita-Kumamoto Tectonic Line) could also be a mere effect of rotation of the arc segment located close to the hinge point. Interestingly enough, Kamata and Kodama [1994] have noted the synchronous occurrence of dextral shearing along the Oita-Kumamoto Tectonic Line, anticlockwise rotation of the northeastern Ryukyu Arc and the formation of rift segments striking oblique to the tectonic lineament.
The Wadati-Benioff zone of the subducting Philippine slab increases in depth from the northeast to the southwest, where it abruptly ends in the southwest just east of Taiwan (Figure 10). This could imply a greater slab length (or at least a colder slab) in the southwest and therefore a greater slab pull force, resulting in faster rollback in the southwest. This increase in retreat is facilitated in the southwest by the discontinuity of the slab, which allows asthenosphere material to flow underneath the slap tip from the ocean side towards the asthenosphere wedge, as well as horizontally around the slab edge. This is not possible in the northeast due to the continuity of the slab across the northeastern cusp. The increase in retreat velocity along the subduction zone is supported by recent GPS data from the southern Ryukyu Arc (Figure 10) [Imanishi et al. 1996; Heki et al. 1996] and could explain the southwestward decrease in crustal thickness of the Okinawa Trough. The abrupt ending of the slab in the southwest seems to represent a vertical tear, where the horizontal projection of this tear is oriented north-south and closely coincides with the steep continental margin of eastern Taiwan, also striking north-south. This leads to the tentative conclusion that whilst the Philippine slab is retreating towards the south to southwest, it is forming a tear between the buoyant (continental) part of the Philippine plate (Taiwan) and the dense (oceanic) part of the Philippine plate. The location of this tear could be triggered by the transition from continental to oceanic lithosphere.
Another example of asymmetrical arc systems is the New Hebrides Arc from the southwest Pacific (Schellart et al. 2002a). This arc system is situated in an ocean-ocean subduction setting and is flanked by the North Fiji Basin, a wedge-shaped active back-arc basin, which developed since ~ 12-11 Ma [Pelletier et al. 1993] due to asymmetrical clockwise opening (Figure 1). In an initial stage of opening, back-arc spreading ridges had a strike ~ parallel to the arc (Figure 1b), but in a later stage this pattern became more complicated with arc-oblique spreading ridges near the hinge point (Figure 1c). The most recent stage of development of the arc and back-arc basin has been complicated by subduction of the d'Entrecasteaux Ridge and the West Torres Plateau.
The Kuril Arc system could also be regarded as a system that has developed to a large extent by asymmetrical rollback. The system is situated in an ocean-continent subduction setting and is flanked by the Kuril Basin and the Sea of Okhotsk, which developed by anticlockwise retreat of the Pacific slab from the Eocene until the Middle Miocene. The back-arc region is characterised by diffuse deformation in the Sea of Okhotsk and a late stage opening of the Kuril Basin, which is thought to be underlain by oceanic crust.
The New Hebrides Arc system and the Kuril Arc system will be discussed more extensively elsewhere [Schellart et al., in press; Schellart et al., submitted].